
Multidimensional Chromatography
.pdfBiomedical and Pharmaceutical Applications |
253 |
analyte enrichment prior to LC analysis. In LC, a popular way towards enhanced selectivity and sensitivity is the use of small solid-phase extraction (SPE) cartridges filled with a more or less selective sorbent for the preconcentration of samples (3). The application of the on-line combination of SPE and LC for bioanalytical purposes will be discussed to some extent in the next section. Another viable approach towards more chromatographic selectivity is coupled-column LC (LC – LC) in which two or more analytical columns are combined in an on-line fashion to accomplish the isolation of the compound(s) of interest. LC – LC can be used either for the profiling of a complete sample or for the analysis of target compounds (4).
11.2.1COMPREHENSIVE LC – LC
The objective of the profiling mode of LC – LC is to fractionate all components of the analysed mixture. This may be accomplished by so-called comprehensive twodimensional LC in which the entire chromatogram eluting from the primary column is submitted to the secondary column. The secondary instrument must operate fast enough to preserve the information contained in the primary signal. That is, it should be able to generate at least one chromatogram during the time required for a peak to elute from the primary column. Until now, a limited number of studies involving comprehensive LC – LC for the analysis of compounds of biological interest have been reported. Most of these studies were carried out within the group of Jorgenson and mainly deal with the design, construction and implementation of comprehensive LC – LC systems for the separation of either a complex protein mixture or an enzymatic digest of a protein (i.e. a mixture of peptides) (5 – 10). For these purposes, orthogonal on-line combinations of ion-exchange chromatography, size-exclusion chromatography (SEC) or reversed-phase (RP) LC are used. In a recent study, peptide fragments generated in the tryptic digests of ovalbumin and serum albumin are separated by SEC in the first dimension (run time, 160 min) and fast RPLC in the second (run time, 240 s) (7). Following RPLC, the peptides flow to an electrospray mass spectrometer for on-line identification. The complete LC system yields a peak capacity of almost 500, thereby maximizing the chance of completely resolving each peptide of the digest and, thus, permitting highly reliable peptide mapping. In addition, a comprehensive ion-exchange LC – RPLC – mass spectrometry (MS) system for the analysis of proteins was demonstrated in which a 120-min ion-exchange LC run is sampled by 48 RPLC runs of 150 s, leading to a peak capacity of over 2500
(8). The system was succesfully applied to the screening of an Escherichia coli lysate without any prior knowledge of the characteristics (e.g. molecular weight, isoelectric point, hydrophobicity, etc.) of its individual components.
Other bioanalytical applications of systems in which the eluate of a first LC column is sampled in continuous and repetitive intervals and subjected to a second LC dimension are, for example, described by Wheatly et al. (11) and Matsuoka et al. (12). Wheatly coupled gradient affinity LC with RPLC for the determination of the isoenzymaticand subunit composition of glutathione S-transferses in cytosol
254 |
Multidimensional Chromatography |
extracts of human lung and liver tissues. Matsuoaka combined anion-exchange LC with RPLC for the analysis of the enzymatic digests of bovine calmodulin and D59 protein, and for the separation of a crude peptide mixture which was extracted from bovine brain tissue.
With comprehensive two-dimensional LC, indeed truly orthogonal separations with very high efficiency and peak capacity can be obtained, although the interfacing can be quite complicated. Additionally, in bioanalysis large peak capacities are often not needed, especially when the objective is the determination of only a few target compounds (see next section). The usefulness of comprehensive LC – LC lies particularly in the entire profiling of protein and peptide mixtures of unknown composition (protein/peptide mapping). In this respect, comprehensive LC – LC might be an alternative for two-dimensional gel electrophoresis, with LC – LC having the advantage that it can be on-line coupled to MS so that molecular-weight information becomes available fast and without the need for analyte transfer.
11.2.2 HEART-CUT LC – LC
In contrast to comprehensive LC – LC, the goal of the targeted mode of multidimensional LC is to isolate a single analyte or small group of components of a complex mixture. Target analysis by LC – LC is normally carried out by using the principle of ‘heart-cutting’. In this approach, an eluting zone from the first LC column (heart-cut) is switched to a second column for subsequent analysis of the transferred compounds. Thus, the first column is used to extract and enrich analytes, e.g. from complex biological fluids, while the second column is used to separate the molecule(s) of interest. This combination leads to an enhanced selectivity and sensitivity, because the target compounds are preconcentrated and/or can be detected in the absence (or reduced presence) of interfering compounds. As a result, lower detection limits are obtained and the reliability of quantitative analysis is strongly improved. When a very high selectivity is required, the dimensionality (number of subsequent columns) may be increased to three or more by using different retention modes. Of course, on-line LC – LC requires the use of mobile phases which, to some extent, are compatible with the columns involved. Therefore, LC – LC is restricted to certain chromatographic combinations, which often include RPLC, ion-exchange, polar bonded-phase, chiral or affinity columns. It is evident that next to the selection of the proper columns and eluents, correct timing of the column switching and optimization of the transfer volume are essential aspects, and, therefore, systematic method development is an important issue in LC – LC (13 – 15).
In biomedical analysis, LC – LC has been used most extensively and successfully in the heart-cut mode for the analysis of drugs and related compounds in matrices such as plasma, serum or urine. Table 11.1 gives an overview of analytes in biological matrices which have been determined by heart-cut LC – LC systems. A typical example of such an approach is the work of Eklund et al. (16) who determined the free concentration of sameridine, an anaesthetic and analgesic drug, in blood plasma

Table 11.1 Biomedical applications of on-line heart-cut LC–LC
|
|
Sample |
First |
Subsequent |
|
|
Analyte(s) |
Sample matrix |
preparationa |
LC modeb |
LC mode(s)b |
Detectionc |
Reference |
|
|
|
|
|
|
|
Drugs and Related Compounds |
|
|
|
|
|
|
Ampicillin |
human plasma |
deproteination |
C18 |
C18 |
post-Flu |
28 |
|
human urine |
buffer dilution |
C18 |
C18 |
post-Flu |
28 |
-agonists |
human/bovine urine |
none |
C18 |
C18 |
UV |
22 |
|
bovine urine |
none |
C18 |
C18 |
MS–MS |
23 |
Basic drugs |
human serum |
liq – liq extraction |
ion-pair C18 |
C18 |
UV |
37 |
Bupivacaine |
human plasma |
ultrafiltration |
C8 |
CIEX |
UV |
24 |
Chloramphenicol |
pig tissue |
liq –liq extraction |
PRP |
C18 |
UV |
32 |
Dexamethasone |
bovine tissue |
liq – liq extraction |
phenyl |
silica; CN |
UV |
29 |
Dopa and its |
human/rat plasma |
deproteination |
CIEX |
C18 |
EC |
66 |
metabolite |
|
|
|
|
|
|
Efletirizine |
human plasma |
SPE |
C18 |
C18 |
UV |
69 |
|
human urine |
dilution |
C18 |
C18 |
UV |
69 |
Glycyrrhizin and its |
human plasma |
SPE |
C18 |
C18 |
UV |
39 |
metabolite |
|
|
|
|
|
|
Ibuprofen |
human serum |
liq – liq extraction |
ion-pair C18 |
C18; C18 |
UV |
34 |
Manidipine and its |
human serum |
liq – liq extraction |
ion-pair C18 |
C18 |
UV |
38 |
metabolite |
|
|
|
|
|
|
Mefenamic acid |
human serum |
liq – liq extraction |
C18 |
C18; C18 |
UV |
34 |
Melengestrol |
bovine tissue |
liq – liq extraction |
phenyl |
silica; silica |
UV |
31 |
acetate |
|
|
|
|
|
|
Applications Pharmaceutical and Biomedical
255
|
|
Table 11.1 |
(continued) |
|
|
|
|
|
|
|
|
|
|
|
|
Sample |
First |
Subsequent |
|
|
Analyte(s) |
Sample matrix |
preparation a |
LC modeb |
LC mode(s) b |
Detection c |
Reference |
|
|
|
|
|
|
|
Methandrostenolone |
equine |
liq – liq extraction |
phenyl |
C18; C8 |
UV and |
30 |
and its metabolites |
plasma/urine |
|
|
|
MS–MS |
|
Metyrapone |
human |
liq – liq extraction |
silica |
Chiralcel OJ |
UV |
42 |
|
plasma/urine |
|
|
|
|
|
Phenyl- |
human |
liq – liq extraction |
ion-pair C18 |
C18 |
UV |
36 |
propanololamine |
plasma/urine |
|
|
|
|
|
Probenicid |
rat plasma |
deproteination |
C18 |
ion-pair C18 |
UV |
63 |
Propanolol |
human plasma |
liq – liq extraction |
ion-pair C18 |
C18 |
UV |
35 |
Methotrexate |
human urine |
SPE |
AIEX |
C18 |
UV |
26 |
Remoxipride |
human plasma |
liq – liq extraction |
CN |
C18 |
EC |
17 |
metabolite |
|
|
|
|
|
|
Ro 23-7637 |
dog plasma |
SPE |
CIEX |
C18 |
UV |
27 |
Ro 24-0238 |
human plasma |
SPE |
CIEX |
C8 |
UV |
25 |
Ropivacaine |
human plasma |
ultrafiltration |
C8 |
CIEX |
UV |
24 |
Sameridine |
human plasma |
ultrafiltration |
C18 |
CIEX; CIEX |
UV |
16 |
TCV-116 and |
human |
liq – liq extraction |
C18 |
C18 |
Flu |
64 |
its metabolites |
serum/urine |
|
|
|
|
|
Tipredane |
rat urine |
none |
CN |
C18 |
UV |
18 |
metabolites |
|
|
|
|
|
|
|
human urine |
none |
C18 |
C1 |
UV |
19 |
Vanillylmandelic |
human urine |
acidification, |
ion-pair C18 |
AIEX |
EC |
65 |
acid |
|
centrifugation |
|
|
|
|
Various drugs |
human |
none |
micel C8 |
C18 |
UV or Flu |
33 |
|
serum/plasma |
|
or CN |
|
|
|
256
Chromatography Multidimensional
Zidovudine- -D- |
rat plasma |
deproteination |
C18 |
ion-pair C18 |
UV |
63 |
glucuronide |
|
|
|
|
|
|
Enantiomers of Drugs and Related Compounds |
|
|
|
|
|
|
Amino acids |
protein hydrolysates, |
various |
CIEX |
Crownpak |
post-Flu |
43 |
|
bacterial cultures, |
|
|
CR( ) |
|
|
|
food, urine |
|
|
|
|
|
Artilide fumarate |
human/anim. |
SPE |
C8 |
C18; Pirkle |
pre-Flu |
44 |
|
plasma |
|
|
|
|
|
Bupivacaine |
human plasma |
liq – liq extraction |
AGP |
C18; C8 |
UV |
54 |
Chlortalidone |
human whole |
liq – liq extraction |
CN |
-CD phenyl |
UV |
51 |
|
blood |
|
|
|
|
|
Dihydropyridine |
dog plasma |
liq –liq extraction |
AIEX |
Ovomucoid |
EC |
49 |
calcium blocker |
|
|
|
|
|
|
Ibutilide fumarate |
human/animal |
SPE |
C8 |
C18; Pirkle |
pre-Flu |
44 |
|
plasma |
|
|
|
|
|
Ifosamide |
human plasma |
liq – liq extraction |
D,L-naphthyl- |
Chiralcel OD |
UV |
55 |
|
|
|
alanine |
|
|
|
Ketoprofen |
human plasma |
deproteination |
C18 |
C18; Ovomucoid |
UV |
47 |
Leucovorine |
dog plasma |
deproteination |
BSA-silica |
C18 |
UV |
53 |
|
human plasma |
deproteination |
phenyl |
BSA-silica |
UV |
56 |
Manidipine |
human serum |
liq – liq extraction |
C18 |
C18 |
UV |
45 |
|
|
Chiralcel OJ |
|
|
|
|
Mefloquine |
human plasma/whole |
liq – liq extraction |
CN |
silica; (S)- |
UV |
52 |
|
blood |
|
|
naphthylurea |
|
|
Metoprolol |
human plasma |
SPE |
AGP |
C18; C18 |
Flu |
54 |
Metyrapol |
human |
liq – liq extraction |
silica |
Chiralcel OJ |
UV |
42 |
|
plasma/urine |
|
|
|
|
|
Applications Pharmaceutical and Biomedical
257
|
|
Table 11.1 |
(continued) |
|
|
|
|
|
|
|
|
|
|
|
|
Sample |
First |
Subsequent |
|
|
Analyte(s) |
Sample matrix |
preparation a |
LC mode b |
LC mode(s) b |
Detection c |
Reference |
|
|
|
|
|
|
|
Oxazepam |
human plasma |
SPE |
BSA-silica |
C18; C18 |
UV |
54 |
p-HPPH d |
rat liver |
liq – liq extraction |
C18 |
C18 with |
UV |
46 |
|
microsomes |
|
|
L-prolinamide |
|
|
Pimobendan and its |
human plasma |
liq – liq extraction |
silica |
Chiralcel OD |
UV |
48 |
metabolite |
|
|
|
|
|
|
Terbutaline |
human plasma |
SPE |
phenyl |
-CD C18 |
EC |
51 |
|
human plasma |
SPE |
AGP |
C18; C18 |
EC |
54 |
|
human plasma |
SPE |
phenyl |
-CD |
EC or MS |
57 |
Verapamil and |
human plasma |
liq – liq extraction |
C18 |
Ovomucoid |
UV |
50 |
its metabolites |
|
|
|
|
|
|
Endogenous Compounds |
|
|
|
|
|
|
Creatinine |
human |
buffer dilution |
SEC |
SEC; CIEX |
UV |
58 |
|
serum urine |
|
|
CIEX; |
|
|
Glutathione |
cytosol of human |
homogenization, |
affinity |
C8 or C18 |
UV |
11 |
S-transferases |
lung tissue |
centrifugation |
|
|
|
|
Hydroxy- |
human urine |
SPE |
PRP |
C18 |
EC |
59 |
deoxyguanosine |
|
|
|
|
|
|
Hydroxytryptamine |
rabbit blood |
centrifugation |
CIEX |
C18 |
EC |
68 |
and its metabolite |
|
on-line SPE |
|
|
|
|
Immunoglobulin G |
bovine serum |
none |
SEC |
affinity |
UV |
60 |
and its multimers |
|
|
|
|
|
|
Neopterin |
human serum |
centrifugation |
C18 |
ion-pair C18 |
Flu |
62 |
Proinsulin fusion |
E. coli cells |
sulfitolysis |
SEC |
AIEX |
UV |
61 |
protein |
|
|
|
|
|
|
Riboflavin |
human |
deproteination |
PRP |
C18 |
Flu |
67 |
|
blood/plasma |
|
|
|
|
|
258
Chromatography Multidimensional

Thiamineand |
human |
deproteination |
PRP |
ion-pair PRP |
post-Flu |
67 |
pyridoxal-phoshates |
blood/plasma |
|
|
|
|
|
Uric acid |
human |
buffer dilution |
SEC |
AIEX; SEC |
UV |
58 |
|
serum/urine |
|
|
|
|
|
aliq – liq, liquid – liquid; SPE, solid-phase extraction.
baffinity, affinity chromatography; AGP, immobilized 1-acid glycoprotein; AIEX, anion-exchange; -CD, -cyclodextrines; BSA, bovine serum albumin; C1, methylsilica; C8, octylsilica; C18, octadecylsilica; CIEX, cation-exchange; Crown pak CR( ), octadecyl silica coated with chiral crown ether; CN, cyanopropylsilica; ion pair, ion-pair chromatography; micel, micellar chromatography; phenyl, phenylsilica; Pirkle, 3,5-dinitrobenzoyl-D-phenylglycine-bonded silica; PRP, polymeric reversed-phase; SEC, size-exclusion chromatography.
cEC, electrochemical detection; Flu, fluorescence detection; MS, mass spectrometric detection; pre-Flu, fluorescence detection after pre-column derivatization; postFlu, fluorescence detection after post-column derivatization; UV, UV absorbance detection.
dp-HPPH, p-hydroxyphenyl phenylhydantoin.
Applications Pharmaceutical and Biomedical
259
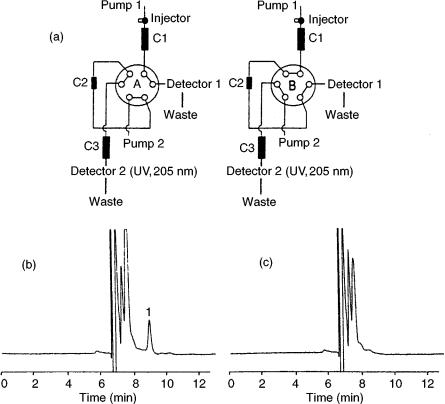
260 |
Multidimensional Chromatography |
by coupling RPLC and ion-exchange LC. After ultrafiltration of the blood sample, 400 l of the ultrafiltrate was injected into an LC – LC system (Figure 11.1(a)) without further pretreatment. A heart-cut (ca. 1 ml) of the eluate of the first column (octadecylsilica (ODS)) which contained the sameridine, was transferred to and enriched on, a cation-exchange extraction column. In the next step, the concentrated sample was desorbed into the second analytical column (cation-exchange) for the final separation and subsequent UV detection at 205 nm. In this way the interfer- ence-free and sensitive determination of sameridine could be achieved (Figures 11.1(b) and (c)) with a limit of quantitation (LOQ) of 1 nM and a within-day precision of 2 – 8 %. In a similar approach, Baker et al. (18) determined the major
Figure 11.1 (a) Schematic representation of a coupled-column LC system for sameridine analysis consisting of a reversed-phase analytical column (C1), a cation-exchange extraction column (C2) and a cation-exchange analytical column (C3); (b) chromatogram of plasma sample after intrathecal administration of sameridine (plasma concentration, 11.2 nM); (c) chromatogram of a blank plasma sample. Reprinted from Journal of Chromatography, B 708, E. Eklund et al. ‘Determination of free concentration of sameridine in blood plasma by uttrafiltration and coupled-column liquid chromatography,’ pp. 195 – 200, copyright 1998, with permission from Elsevier Science.

Biomedical and Pharmaceutical Applications |
261 |
metabolite of the glucocorticoid tipredane in rat urine which was injected directly into the first LC column. In this case, the initial separation was performed on a cyanopropylsilica column and a portion of the eluate was switched to an ODS column where the final separation was carried out. This method allowed quantification of the metabolite down to 25 ng/ml, showed acceptable linearity, precision and accuracy in the 25 – 5000 ng/ml range, and was successfully used for a long-term toxicology study. Lanbeck-Vallén et al. (28) used post-column derivatization with fluorescamine for the analysis of the penicillin ampicillin in plasma by LC with fluorescence detection. However, since fluorescamine reacts with most primary amines, the selectivity of the LC system had to be increased. This was achieved by the use of a coupled column system (Figure 11.2(a)). The total combination yielded a highly selective system which permitted the sensitive determination of ampicillin (Figure 11.2(b)) with a detection limit of 14 nM for 0.5 ml plasma samples.
Figure 11.2 (a) LC – LC system with post-column reaction detection for the determination of ampicillin in plasma; (b) Chromatogram of plasma sample (collected 10 min after oral administration of 670 mol of ampicillin) containing 1.26 M ampicillin (amp). Reprinted from Journal of Chromatography, 567, K. Lanbeck-Vallén et al., ‘Determination of ampicillin in biological fluids by coupled-column liquid chromatography and post-column derivatization,’ pp. 121 – 128, copyright 1991, with permission from Elsevier Science.
262 |
Multidimensional Chromatography |
In its true sense, the term ‘multidimensional’ refers to LC systems in which there is a distinct difference in retention mechanisms in the columns used. Still, column switching without change in retention mode can be a relatively easy to implement approach for separating complex mixtures. This is a practical substitute for lineargradient elution and is particularly useful for analyte enrichment and sample cleanup, as has been extensively demonstrated by Hoogendoorn and co-workers (14, 20, 21) for environmental analysis. The concept is also useful in bioanalysis, as was shown by Polettini et al. (22) who applied coupled-column RPLC using two identical ODS columns for the automated analysis of -agonists in urine samples. With direct injection of large volumes (1.5 ml) of human urine and careful adjustment of transfer volumes and mobile phase conditions, LC – LC with UV detection allowed the determination of clenbuterol at the low-ng/ml level. In a subsequent study (23), the RPLC – RPLC system was coupled to a tandem MS system in order to gain further selectivity and sensitivity. With this set-up, -agonists could be analysed in bovine urine with a LOQ of 0.1 ng/ml. For the determination of clenbuterol at the 1 ng/ml level, the inter-day reproducibility was 8.4 %. With similar hydrophobic columns, still different retention modes can be established by using one in the ionpairing mode and the other in the RP mode. This approach has been used by Yamashita and co-workers (34 – 38) for the analysis of basic and acidic drugs in biological fluids. For example, phenylpropanololamine was determined in human plasma and urine by first pre-separating the basic drug from endogenous compounds on an ODS column using a low-pH eluent containing butanesulfonate as the ion-pair reagent. Subsequently, after column switching of the heart-cut containing the analyte, further separation is carried out on a second, identical column in the absence of butanesulfonate. The selectivity of the method was such that short-wavelength UV detection could be used, thus yielding a detection limit of 0.4 ng/ml in plasma. The method was applied to the determination of phenylpropanololamine in the plasma of human volunteers after oral administration of 25 mg of the drug.
The on-line coupling of achiral and chiral columns is now a proven concept for the bioanalytical separation of enantiomers (40, 41). Table 11.1 summarizes a number of these LC – LC applications. Frequently, first an achiral column is used to separate enantiomeric pairs from matrix components, and then the enantiomers are transferred to a chiral column for selective separation (Figure 11.3). In this way, the chiral separation is often enhanced by excluding interfering substances from the second column. Van de Merbel et al. (43) used two-dimensional LC for the determination of D- and L-enantiomers of amino acids in biological samples. The amino acids are first separated by ion-exchange chromatography and subsequent enantioseparation is achieved by injection of 3 l heart-cuts on to a second column with a chiral crown ether stationary phase. The selectivity and sensitivity of the system was further increased by using fluorescence detection after on-line post-column labelling of the amino acids with o-phthalaldehyde. In this way, small quantities of D-enan- tiomers could be determined in complex biological samples such as protein hydrolysates, urine, bacterial cultures and yoghurt, with hardly any additional pretreatment. Another typical example of achiral – chiral coupled LC is the