
Physics of biomolecules and cells
.pdf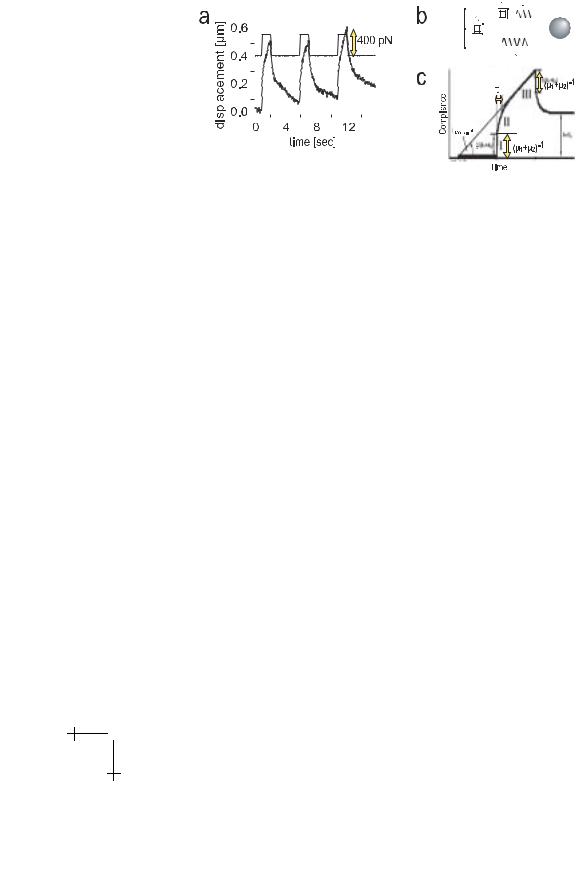
E. Sackmann et al.: Physics of Composite Cell Membranes |
269 |
|||||||||||||||||||||||||||||||||
|
|
|
|
|
|
|
|
|
|
|
|
|
|
|
|
|
|
|
|
|
|
|
|
|
|
|
|
|
|
|
|
|
|
|
|
|
|
|
|
|
|
|
|
|
|
|
|
|
|
|
|
|
|
|
|
|
|
|
|
|
|
|
|
|
|
|
|
|
|
|
|
|
|
|
|
|
|
|
|
|
|
|
|
|
|
|
|
|
|
|
|
|
|
|
|
|
|
|
|
|
|
|
|
|
|
|
|
|
|
|
|
|
|
|
|
|
|
|
|
|
|
|
|
|
|
|
|
|
|
|
|
|
|
|
|
|
|
|
|
|
|
|
|
|
|
|
|
|
|
|
|
|
|
|
|
|
|
|
|
|
|
|
|
|
|
|
|
|
|
|
|
|
|
|
|
|
|
|
|
|
|
|
|
|
|
|
|
|
|
|
|
|
|
|
|
|
|
|
|
|
|
|
|
|
|
|
|
|
|
|
|
|
|
|
|
|
|
|
|
|
|
|
|
|
|
|
|
|
|
|
|
|
|
|
|
|
|
|
|
|
|
|
|
|
|
|
|
|
|
|
|
|
|
|
|
|
|
|
|
|
|
|
|
|
|
|
|
|
|
|
|
|
|
|
|
|
|
|
|
|
|
|
|
|
|
|
|
|
|
|
|
|
|
|
|
|
|
|
|
|
|
|
|
|
|
|
|
|
|
|
|
|
|
|
|
|
|
|
|
|
|
|
|
|
|
|
|
|
|
|
|
|
|
|
|
|
|
|
|
|
|
|
|
|
|
|
|
|
|
|
|
|
|
|
|
|
|
|
|
|
|
|
|
|
|
|
|
|
|
|
|
|
|
|
|
|
|
|
|
|
|
|
|
|
|
|
|
|
|
|
|
|
|
|
|
|
|
|
|
|
|
|
|
|
|
|
|
|
|
|
|
|
|
|
|
|
|
|
|
|
|
|
|
|
|
|
|
|
|
|
|
|
|
|
|
|
|
|
|
|
|
|
|
|
|
|
|
|
|
|
|
|
|
|
|
|
|
|
|
|
|
|
|
|
|
|
|
|
|
|
|
|
|
|
|
|
|
|
|
|
|
|
|
|
|
|
|
|
|
|
|
|
|
|
|
|
|
|
|
|
|
|
|
|
|
|
|
|
|
|
|
|
|
|
|
|
|
|
|
|
|
|
|
|
|
|
|
|
|
|
|
|
|
|
|
|
|
|
|
|
|
|
|
|
|
|
|
|
|
|
|
|
|
|
|
|
|
|
|
|
|
|
|
|
|
|
|
|
|
|
|
|
|
|
|
|
|
|
|
|
|
|
|
|
|
|
|
|
|
|
|
|
|
|
|
|
|
|
|
|
|
|
|
|
|
|
|
|
|
|
|
|
|
|
|
|
|
|
|
|
|
|
|
|
|
|
|
|
|
|
|
|
|
|
|
|
|
|
|
|
|
|
|
|
|
|
|
|
|
|
|
|
|
|
|
|
|
|
|
|
|
|
|
|
|
|
|
|
|
|
|
|
|
|
|
|
|
|
|
|
|
|
|
|
|
|
|
|
|
|
|
|
|
|
|
|
|
|
|
Fig. 15. a) Typical viscoelastic response curve of magnetic bead in cytoplasm of macrophage. Obtained for 1 µm diameter magnetic bead and f ≈ 400 pN. Note that J (t) is composed of three regimes: a fast elastic response (I), a relaxation regime (II) and a viscous flow (III). b) Mechanical equivalent circuit enabling formal representation of creep response curve of cytoplasm of macrophages. The dashpot η0 accounts for the viscous flow due to the viscoplasticity of cell. The e ective total spring constant is µ = µ1 + µ2. c) Typical response function calculated for the Zener model as given in equation (4.1).
and is a measure for the time interval within which the local bonds yield resulting in the flow of the bead.
In reality the relaxation process is determined by a distribution of relaxation times and a more general expression for the deflection x (t) would be
x (t) = F |
i |
Ji |
1 − exp |
−τi |
+ |
η |
(4.2) |
|
|
|
|
|
|
t |
|
t |
|
where Ji = ki−1 are elastic compliances characterizing the softness of internal bonds.
The viscoelastic parameters of the mechanical model are relative measures for the membrane sti ness and viscosity. In order to obtain true elastic constants and viscosities of the cell surface one has to analyze the viscoelastic response in terms of the theory of the elasticity of shells. This is an unsolvable problem for large heterogeneously structured shells. Fortunately, measurements of the deformation field evoked within the membrane by a local tangential force showed that the membrane deformation is a local quantity. This deformation field mapping technique is illustrated in Figure 16. It is based on the analysis of the deflection of an assembly of small non-magnetic beads which are anchored to the cell surface together with the magnetic tweezers. It is seen (cf. Fig. 15b) that the deflection of

270 |
Physics of Bio-Molecules and Cells |
|
||||||
|
|
|
|
|
|
|
|
|
|
|
|
|
|
|
|
|
|
|
|
|
|
|
|
|
|
|
|
|
|
|
|
|
|
|
|
|
|
|
|
|
|
|
|
|
|
|
|
|
|
|
|
|
|
|
|
|
|
|
|
|
|
|
|
|
|
|
|
|
|
|
|
|
|
|
|
|
|
|
|
|
|
|
|
|
|
|
|
|
|
|
|
|
|
|
|
|
|
|
|
|
|
|
|
|
|
|
|
Fig. 16. a) Deformation field mapping technique. For the measurement of the range of the elastic strain field u (r) generated by a tangential force a magnetic bead is coupled to integrin receptors αV β3 through fibronectin and non-magnetic beads are coupled to the cell surface by nonspecific (electrostatic) forces or fi- bronectin. The tangential deflection u (r) of these colloidal probes induced by lateral deflection of the magnetic tweezer is measured as a function of distance of the probe from the center of the applied force f0. Mechanical model of thin lobe of cell which corresponds to the situation of spread fibroblasts or endothelial cells. Each of the two juxtaposed membranes are coupled to dense actin network and these are interconnected by inner cytoskeleton. b) Plot of lateral displacement ux (r) in the direction (x) of the force as a function of the distance from the force as predicted by the theory of deformation of a thin plate coupled to an underlying network which is fixed to the surface. The range of the decay field and the elastic shear modulus is obtained by fitting the theoretical curve u (r) to the experimental data.
the non-magnetic beads, u (r), decays rather rapidly with the distance from the source of the force. The range of the deformation is characterized by a decay length of λ−1 5−10 µm, depending on the cell type. This is a very important result since it shows that the elasticity of composite cell membranes is a local quantity. A detailed theoretical analysis shows that the decay of the deformation field (induced by a point force) with the distance can be explained in terms of the coupling of the lipid/protein bilayer to the intracellular sca old. The deformation of a thin plate coupled to a network (fixed to a substrate) is determined by the following di erential equation
∆u + |
1 |
+ σ |
u − λ2u = − |
F |
, |
(4.3) |
||
|
|
|
|
|
||||
1 |
− |
σ |
µ |
|
||||
|
|
|
|
|
|
|
where µ is the two-dimensional shear modulus which is related to the bulk modulus µ by µ = µh, where h is the membrane thickness. The parameter λ has the dimension of a reciprocal length and λ−1 is thus a measure

E. Sackmann et al.: Physics of Composite Cell Membranes |
271 |
for the screening length. It is determined by the coupling strength of the bilayer membrane to the underlying sca old. This coupling strength can be expressed in terms of a spring constant per unit area (χ = J/m4) and since λ−2 = µ /χ, measurements of the screening length yield insight into the coupling strength of the clustered integrin receptors to the actin cortex. This is still a rough model and more refined microscopic theories of composite cell membranes are required to derive reliable viscoelastic moduli from microrheological studies. In particular it is essential to separate passive and active viscoelastic responses as shown in Section 6.
5Activation of endothelial cells: On the possibility of formation
of stress fibers as phase transition of actin-network triggered by cell signalling pathways
The centripetal contraction of endothelial cells required for the formation of gaps within the confluent endothelial cell larger lining the inner wall of blood vessels involves two processes. First the formation of actin stress fibers within or close to the initially random network of the cortex (cf. also Fig. 3) and second, the aggregation and activation of myosin II to form acto-myosin motors.
The microscopic details of this process are still largely obscure, but there is ample evidence that the structural change in the actin cortex and the activation of the myosin motor is mediated by intracellular signal transduction involving GTP-hydrolyzing proteins so called G-proteins. Several signal pathways are known which regulate the organization and functionality of the actin sca old on di erent time scales.
For biophysical studies the process of endothelial cell contraction can be induced by the inflammation agents thrombin and histamine which allow to mimick the gap opening under controlled conditions. Monolayers of endothelial cells from veins of human naval cord are grown on solid supports. Addition of above mentioned thrombin or histamine induces dramatic e ects. Firstly, it causes the formation of numerous actin bundles within the actin cortex within a few seconds, which can be visualized by labelling actin with fluorescent derivatives of phalloidin (cf. Fig. 17b). Secondly, the stimulation causes centripetal contraction of the cells by disrupture of cadherin-cadherin bonds resulting in the formation of gaps within the initially confluent cell monolayer. This gap formation can be detected with very high sensitivity through the reduction of the electrical impedance measured by electrical impedance spectroscopy (cf. Wegener et al. 1999; Hillebrandt et al. 2001). Thirdly, the shear elastic modulus of the composite membrane increases dramatically. As demonstrated in Figure 17a this sti ening e ect can be followed and analyzed in real time by repeated
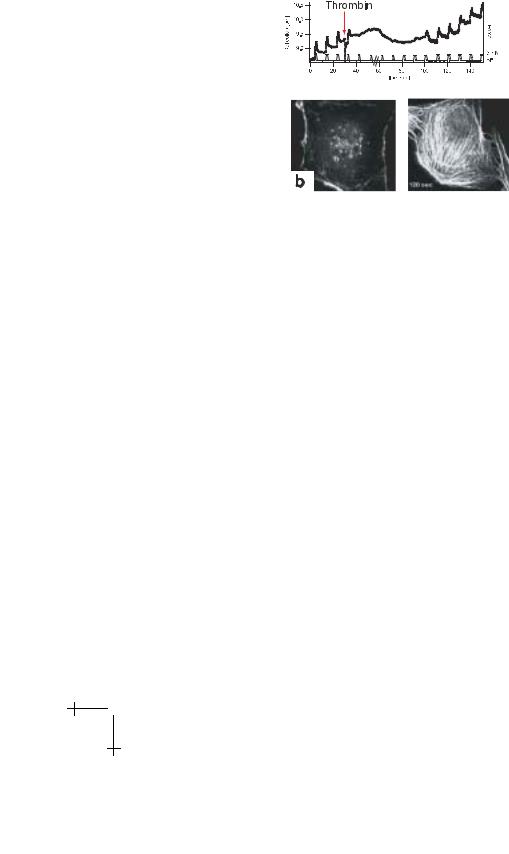
272 |
Physics of Bio-Molecules and Cells |
a
Fig. 17. A real time demonstration of rapid formation of stress fibers associated with dramatic sti ening of composite membranes by magnetic tweezer force spectroscopy. A sequence of viscoelastic response curves evoked by force pulses (amplitude f◦ = 2 nN) is recorded to establish the reproducibility of the measurement. The response curves exhibit a fast response, a relaxation regime and finally flow-like behaviour similar to Figure 15 and are fully reversible. Thrombin is added at the time indicated by an arrow. The deflection amplitude decays within 2−5 s after addition of thrombin, but recovers after a few minutes, although thrombin is still present. As demonstrated by the fluorescence micrographs at the bottom the sti ening is accompanied by formation of stress fibers. Note that the actin forms a randomly organized network before addition of thrombin.
observation of local deflections of the magnetic tweezers coupled to integrins αVβ1 through fibronectin. Even forces of up to 10 nN are too weak to evoke appreciable deflections a few seconds after addition of thrombin. The sti - ening e ect relaxes after about 1 hour despite of the continuous presence of thrombin which is most likely due to the downregulation of the thrombin receptor. The close correlation between actin bundling and membrane sti ening strongly suggests that the sti ening is due to the coupling of the stress-fibers to the integrin clusters.
Following Garcia & Schaphorst (1995) the formation of stress fibers, the membrane sti ening and the cell contraction is associated with the three following biochemical processes: (i) the F-actin concentration increases by 60% (most likely due to inhibition of sequestering molecules), (ii) the intracellular Ca++ level increases also by about 50% and (iii) the light chains of myosin II are phosphorylated. Interestingly, in the above described sti - ening response a di erent biochemical pathway is involved: the specific

E. Sackmann et al.: Physics of Composite Cell Membranes |
273 |
|||||||||||||||||||||||||||||||||||||||||||||
|
|
|
|
|
|
|
|
|
|
|
|
|
|
|
|
|
|
|
|
|
|
|
|
|
|
|
|
|
|
|
|
|
|
|
|
|
|
|
|
|
|
|
|
|
|
|
|
|
|
|
|
|
|
|
|
|
|
|
|
|
|
|
|
|
|
|
|
|
|
|
|
|
|
|
|
|
|
|
|
|
|
|
|
|
|
|
|
|
|
|
|
|
|
|
|
|
|
|
|
|
|
|
|
|
|
|
|
|
|
|
|
|
|
|
|
|
|
|
|
|
|
|
|
|
|
|
|
|
|
|
|
|
|
|
|
|
|
|
|
|
|
|
|
|
|
|
|
|
|
|
|
|
|
|
|
|
|
|
|
|
|
|
|
|
|
|
|
|
|
|
|
|
|
|
|
|
|
|
|
|
|
|
|
|
|
|
|
|
|
|
|
|
|
|
|
|
|
|
|
|
|
|
|
|
|
|
|
|
|
|
|
|
|
|
|
|
|
|
|
|
|
|
|
|
|
|
|
|
|
|
|
|
|
|
|
|
|
|
|
|
|
|
|
|
|
|
|
|
|
|
|
|
|
|
|
|
|
|
|
|
|
|
|
|
|
|
|
|
|
|
|
|
|
|
|
|
|
|
|
|
|
|
|
|
|
|
|
|
|
|
|
|
|
|
|
|
|
|
|
|
|
|
|
|
|
|
|
|
|
|
|
|
|
|
|
|
|
|
|
|
|
|
|
|
|
|
|
|
|
|
|
|
|
|
|
|
|
|
|
|
|
|
|
|
|
|
|
|
|
|
|
|
|
|
|
|
|
|
|
|
|
|
|
|
|
|
|
|
|
|
|
|
|
|
|
|
|
|
|
|
|
|
|
|
|
|
|
|
|
|
|
|
|
|
|
|
|
|
|
|
|
|
|
|
|
|
|
|
|
|
|
|
|
|
|
|
|
|
|
|
|
|
|
|
|
|
|
|
|
|
|
|
|
|
|
|
|
|
|
|
|
|
|
|
|
|
|
|
|
|
|
|
|
|
|
|
|
|
|
|
|
|
|
|
|
|
|
|
|
|
|
|
|
|
|
|
|
|
|
|
|
|
|
|
|
|
|
|
|
|
|
|
|
|
|
|
|
|
|
|
|
|
|
|
|
|
|
|
|
|
|
|
|
|
|
|
|
|
|
|
|
|
|
|
|
|
|
|
|
|
|
|
|
|
|
|
|
|
|
|
|
|
|
|
|
|
|
|
|
|
|
|
|
|
|
|
|
|
|
|
|
|
|
|
|
|
|
|
|
|
|
|
|
|
|
|
|
|
|
|
|
|
|
|
|
|
|
|
|
|
|
|
|
|
|
|
|
|
|
|
|
|
|
|
|
|
|
|
|
|
|
|
|
|
|
|
|
|
|
|
|
|
|
|
|
|
|
|
|
|
|
|
|
|
|
|
|
|
|
|
|
|
|
|
|
|
|
|
|
|
|
|
|
|
|
|
|
|
|
|
|
|
|
|
|
|
|
|
|
|
|
|
|
|
|
|
|
|
|
|
|
|
|
|
|
|
|
|
|
|
|
|
|
|
|
|
|
|
|
|
|
|
|
|
|
|
|
|
|
|
|
|
|
|
|
|
|
|
|
|
|
|
|
|
|
|
|
|
|
|
|
|
|
|
|
|
|
|
|
|
|
|
|
|
|
|
|
|
|
|
|
|
|
|
|
|
|
|
|
|
|
|
|
|
|
|
|
|
|
|
|
|
|
|
|
|
|
|
|
|
|
|
|
|
|
|
|
|
|
|
|
|
|
|
|
|
|
|
|
|
|
|
|
|
|
|
|
|
|
|
|
|
|
|
|
|
|
|
|
|
|
|
|
|
|
|
|
|
|
|
|
|
|
|
|
|
|
|
|
|
|
|
|
|
|
|
|
|
|
|
|
|
|
|
|
|
|
|
|
|
|
|
|
|
|
|
|
|
|
|
|
|
|
|
|
|
|
|
|
|
|
|
|
|
|
|
|
|
|
|
|
|
|
|
|
|
|
|
|
|
|
|
|
|
|
|
|
|
|
|
|
|
|
|
|
|
|
|
|
|
|
|
|
|
|
|
|
|
|
|
|
|
|
|
|
|
|
|
|
Fig. 18. Biochemical changes induced by thrombin in endothelial cells according to Garcia et al. (1995). Concerted time dependent increase of intracellular Ca++-concentration, F-actin content and amount of myosin II activated by phosphorylation of the light chain.
inhibition of parts of the described pathway did not result in an inhibition of the sti ening response. This demonstrates that the quantification of the viscoelastic properties is also a powerful tool to study in real time the biochemical process in living cells.
The growth rate of actin filaments is typically 1 µm s−1 and it is hard to imagine that the rapid formation of rather regular arrays of stress fibers is caused by concerted growth of single filaments. In view of the above results, the spontaneous and rapid formation of stress fibers could also be explained in terms of a phase transition of the actin-network if it exists in a state close to the percolation transition. According to the phase diagram of Figure 12a transition from a nearly homogeneous network to a state where the network coexists with actin bundles can be induced both by increasing the F-actin content and/or by activation of the cross-linkers.
It is not known yet whether actin cross-linkers are also activated by thrombin or wether bundling is solely due to the increase of F-actin. In fact, microbundles of myosin could be involved in the actin bundling process as demonstrated in the authors laboratory (M. Keller, authors laboratory, unpublished data). The activation of myosin II by MLC-phosphorylation could thus simultaneously serve the bundling of actin and the formation of contractile fibers. The formation of myosin II microbundles by phosphorylation has been demonstrated for Dictyostelia cells. However in this case the phosphorylation site is located at the heavy long arm (cf. Pasternak et al. 1998; Jungbluth et al. 1994).

274 |
|
|
|
|
|
|
|
|
|
|
|
|
Physics of Bio-Molecules and Cells |
||||||||||||||||
|
|
|
|
|
|
|
|
|
|
|
|
|
|
|
|
|
|
|
|
|
|
|
|
|
|
|
|
|
|
|
|
|
|
|
|
|
|
|
|
|
|
|
|
|
|
|
|
|
|
|
|
|
|
|
|
|
|
|
|
|
|
|
|
|
|
|
|
|
|
|
|
|
|
|
|
|
|
|
|
|
|
|
|
|
|
|
|
|
|
|
|
|
|
|
|
|
|
|
|
|
|
|
|
|
|
|
|
|
|
|
|
|
|
|
|
|
|
|
|
|
|
|
|
|
|
|
|
|
|
|
|
|
|
|
|
|
|
|
|
|
|
|
|
|
|
|
|
|
|
|
|
|
|
|
|
|
|
|
|
|
|
|
|
|
|
|
|
|
|
|
|
|
|
|
|
|
|
|
|
|
|
|
|
|
|
|
|
|
|
|
|
|
|
|
|
|
|
|
|
|
|
|
|
|
|
|
|
|
|
|
|
|
|
|
|
|
|
|
|
|
|
|
|
|
|
|
|
|
|
|
|
|
|
|
|
|
|
|
|
|
|
|
|
|
|
|
|
|
|
|
|
|
|
|
|
|
|
|
|
|
|
|
|
|
|
|
|
|
|
|
|
|
|
|
|
|
|
|
|
|
|
|
|
|
|
|
|
|
|
|
|
|
|
|
|
|
|
|
|
|
|
|
|
|
|
|
|
|
|
|
|
|
|
|
|
|
|
|
|
|
|
|
|
|
|
|
|
|
|
|
|
|
|
|
|
|
|
|
|
|
|
|
|
|
|
|
|
|
|
|
|
|
|
|
|
|
|
|
|
|
|
|
|
|
|
|
|
|
|
|
|
|
|
|
|
|
|
|
|
|
|
|
|
|
|
|
|
|
|
|
|
|
|
|
|
|
|
|
|
|
|
|
|
|
|
|
|
|
|
|
|
|
|
|
|
|
|
|
|
|
|
|
|
|
|
|
|
|
|
|
|
|
|
|
|
|
|
|
|
|
|
|
|
|
|
|
|
|
|
|
|
|
|
|
|
|
|
|
|
|
|
|
|
|
|
|
|
|
|
|
|
|
|
|
|
|
|
|
|
|
|
|
|
|
|
|
|
|
|
|
|
|
|
|
|
|
|
|
|
|
|
|
|
|
|
|
|
|
|
|
|
|
|
|
|
|
|
|
|
|
|
|
|
|
|
|
|
|
|
|
|
|
|
|
|
|
|
|
|
|
|
|
|
|
|
|
|
|
|
|
|
|
|
|
|
|
|
|
|
|
|
|
|
|
|
|
|
|
|
|
|
|
|
|
|
|
|
|
|
|
|
|
|
|
|
|
|
|
|
|
|
|
|
|
|
|
|
|
|
|
|
|
|
|
|
|
|
|
|
|
|
|
|
|
|
|
|
|
|
|
|
|
|
|
|
|
|
|
|
|
|
|
|
|
|
|
|
|
|
|
|
|
|
|
|
|
|
|
|
|
|
|
|
|
|
|
|
|
|
|
|
|
|
|
|
|
|
|
|
|
|
|
|
|
|
|
|
|
|
|
|
|
|
|
|
|
|
|
|
|
|
|
|
|
|
|
|
|
|
|
|
|
|
|
|
|
|
|
|
|
|
|
|
|
|
|
|
|
|
|
|
|
|
|
|
|
|
|
|
|
|
|
|
|
|
|
|
|
|
|
|
|
|
|
|
|
|
|
|
|
|
|
|
|
|
|
|
|
|
|
|
|
|
|
|
|
|
|
|
|
|
|
|
|
|
|
|
|
|
|
|
|
|
|
|
|
|
|
|
|
|
|
|
|
|
|
|
|
|
|
|
|
|
|
|
|
|
|
|
Fig. 19. a) Phase contrast micrograph of intracellular active motion of magnetic beads of 1.4 µm and 0.5 µm diameter in Dictyostelia Discoideum cells. The trajectories consist of nearly straight paths interrupted by local random walks. Note that small beads move first towards the center and then back to the periphery. Since the path is several µm long it occurs most likely along microtubuli. b) Time sequence of velocity of large (top) and small phagosomes (bottom). The peak velocities indicated by arrows ( 2 µm/s for the large bead and 4 µm/s for the small bead) correspond to motions along straight paths. The velocities v ≤ 1 µm/s are mostly found for the local motions.
6 On cells as adaptive viscoplastic bodies
As pointed out above, the cytoplasm is a highly heterogeneous viscoelastic body which must combine high mechanical stability with local softness to enable e ective transport of compartments along the microtubuli and actin filaments by forces of the order of ten piconewton. As demonstrated above such behaviour can be realised by heterogeneous gels consisting of entangeled bundles or clusters of tightly crosslinked fibrous macromolecules interconnected by a few thin bundles in such a way that a fully percolated network results. The magnetic bead microrheometry provides a noninvasive technique to measure the distribution of viscoelastic moduli in cells (cf. Bausch 1999). A convenient and most natural way to incorporate magnetic beads is uptake by phagocytosis. The engulfed beads are wrapped by the plasma membrane with the inner leaflet of the membrane exposed to the cytoplasm. These “magnetosomes” behave then similar to intracellular particles. Two types of studies can be performed: firstly, measurements of intracellular transport forces and secondly, determinations of spatial distributions of the intracellular viscoelastic parameters (shear moduli and friction coe cients) by creep experiments. As an instructive example we consider measurements in Dictyostelium Discoideum cells (cf. Feneberg et al. 2001). Figure 19a shows that the magnetosomes are transported over

E. Sackmann et al.: Physics of Composite Cell Membranes |
275 |
large distances within the cell. This transport occurs often repeatedly from the periphery to the center and back and the bead is finally expelled again. Obviously, the trajectories of the beads consist of nearly straight stretches and local random walks. The local velocities along the quasi straight pathes depend on the bead size and are 4 µm/s for the 0.5 µm and about 2 µm/s for the 1.4 µm bead. The velocity of the local random motion varies between 0.1 µm/s and 0.5 µm/s and does not depend appreciably on the size of the beads.
These findings show that the cytoplasm consists of alternating soft and hard regions. In the soft regions the particles can be transported by the molecular motors over long distances (most likely along microtubuli by kinesin or dynein) while they are trapped in the hard regions. Since the velocity does not depend on the bead size the nearly random local walks are most likely due to actively driven local motions of the cytoplasm which is also directly visible by local motion of intracellular particles (e.g. vacuolea) using phase contrast microscopy. A very remarkable result is that for cells in which myosin II has been knocked out by mutation the velocity distribution of the small local motion is shifted to lower values. This interesting result suggests that the motor protein acts as softener of the cytoskeleton (if ATP is present).
The local viscoelastic response of the cytoplasm of Dictyostelia cells exhibits very complex often non-linear behaviour. Two characteristic response curves are shown in Figure 19. Under the action of a strong shear stress (σ 100 Pa) the bead is deflected with rapid initial velocity and saturates after a relaxation regime. After turning o the stress it relaxes, however, only partially showing that the bead exhibited viscous flow. For a weak stress (σ 50 Pa) the bead does not respond appreciably for the first three seconds and is then suddenly deflected by 2 µm before the deformation saturates. Again, after the the application of the shear stress stops, the bead relaxes only partially, indicating a viscous loss. For higher stresses (σ > 100 Pa) the bead motion does not saturate and it moves over long distances with force-dependent velocity. Analysis of the numerous response curves showed that the yield strength of the cytoplasm varies between 10 and 20 Pa and the apparent cytoplasmic viscosity varies between 10 and 350 Pa·s. This strongly force-dependent behaviour suggests that the cytoplasm behaves as viscoplastic body exhibiting highly heterogeneous yield forces.
It further suggests tat the bead deflection with constant velocity v is a consequence of the breakage of internal bonds rather that the internal friction associated with momentum transfer between the bead and the fluid cytoplasm. It is thus more realistic to characterize the constant bead motion
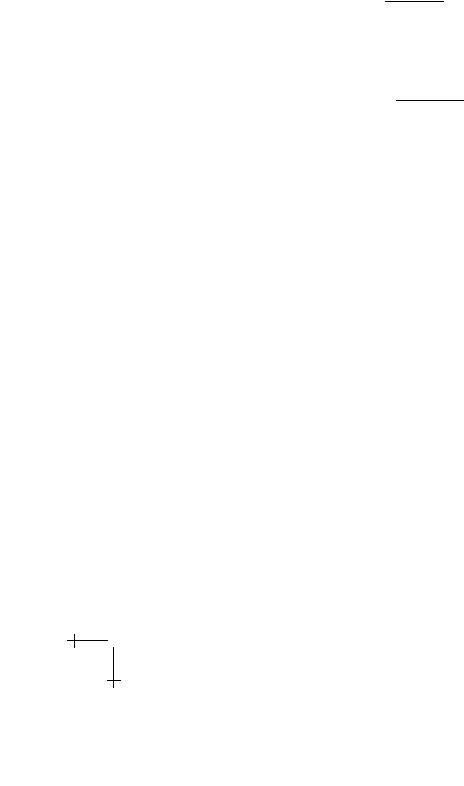
276 |
Physics of Bio-Molecules and Cells |
in terms of a force-dependent local mobility µ (fex) according to:
v = µ (fex) fex. |
|
(6.1) |
|||
Each bond breaks with a rate |
|
|
|
|
|
0 |
|
kBT |
|
||
k = k |
exp |
|
∆g − fexξ |
, |
(6.2) |
|
|
where ∆g is the activation energy of the bond breakage, fexξ is the work associated with the bond breakage and ξ is of the order of the local mesh size. The mobility is then related to the local shear stress σ by
µ (f |
|
) = µ exp |
|
∆G − ξ2σex |
, |
(6.3) |
|
ex |
0 |
kBT |
|
|
where ∆G is the activation energy of all bonds which must be broken to advance the bead by a distance ξ. For weak external stresses (σ ≤ 50 Pa, Fig. 20, bottom) the deflection scenario depends sensitively on the local yield strength of the cytoplasm. If the latter is smaller than the externally applied stress σex one observes a similar response curve as for the high force. If the
bead is initially embedded in a region of higher yield stress (σyield > σex) only a small deflection is initially observed. It escapes, however, in general
from the pinched state after prolonged application of the force and moves fast again, once a softer region is reached.
Note that within the scope of the viscoplastic model the viscosity is replaced by a reciprocal mobility. Despite of the complex viscoelastic behaviour one can measure distributions of the shear elastic moduli. The range of shear elastic moduli depends on the type of cells. For Dictyostelia Discoideum cells the values vary between 10 Pa ≤ µ ≤ 100 Pa while for macrophages values range from µ = 50 Pa to 600 Pa.
The viscoplastic behaviour is expected to play an important role for the intracellular transport of particles by relatively weak active forces as will be argued in the following. Magnetic tweezers can be applied as force transducers to measure these active transport forces in cells. For this purpose the local motion of magnetosomes is perturbed by application of a force pulse and the active force is obtained by analysing the change of the velocity.
The initial velocity is vact = fact/ζloc where ζloc is the local and unknown frictional coe cient. The changed velocity is
v = vact + fex/ζloc |
(6.4) |
|
|
and the active force is thus obtained from the resulting velocity v during the pulse according to
fact = ζlocv − fex. |
(6.5) |

|
|
E. Sackmann et al.: Physics of Composite Cell Membranes |
277 |
||||||||||||||||||||
|
|
|
|
|
|
|
|
|
|
|
|
|
|
|
|
|
|
|
|
|
|
|
|
|
|
|
|
|
|
|
|
|
|
|
|
|
|
|
|
|
|
|
|
|
|
|
|
|
|
|
|
|
|
|
|
|
|
|
|
|
|
|
|
|
|
|
|
|
|
|
|
|
|
|
|
|
|
|
|
|
|
|
|
|
|
|
|
|
|
|
|
|
|
|
|
|
|
|
|
|
|
|
|
|
|
|
|
|
|
|
|
|
|
|
|
|
|
|
|
|
|
|
|
|
|
|
|
|
|
|
|
|
|
|
|
|
|
|
|
|
|
|
|
|
|
|
|
|
|
|
|
|
|
|
|
|
|
|
|
|
|
|
|
|
|
|
|
|
|
|
|
|
|
|
|
|
|
|
|
|
|
|
|
|
|
|
|
|
|
|
|
|
|
|
|
|
|
|
|
|
|
|
|
|
|
|
|
|
|
|
|
|
|
|
|
|
|
|
|
|
|
|
|
|
|
|
|
|
|
|
|
|
|
|
|
|
|
|
|
|
|
|
|
|
|
|
|
|
|
|
|
|
|
|
|
|
|
|
|
|
|
|
|
|
|
|
|
|
|
|
|
|
|
|
|
|
|
|
|
|
|
|
|
|
|
|
|
|
|
|
|
|
|
|
|
|
|
|
|
|
|
|
|
|
|
|
|
|
|
|
|
|
|
|
|
|
|
|
|
|
|
|
|
|
|
|
|
|
|
|
|
|
|
|
|
|
|
|
|
|
|
|
|
|
|
|
|
|
|
|
|
|
|
|
|
|
|
|
|
|
|
|
|
|
|
|
|
|
|
|
|
|
|
|
|
|
|
|
|
|
|
|
|
|
|
|
|
|
|
|
|
|
|
|
|
|
|
|
|
|
|
|
|
|
|
|
|
|
|
|
|
|
|
|
|
|
|
|
|
|
|
|
|
|
|
|
|
|
|
|
|
|
|
|
|
|
|
|
|
|
|
|
|
|
|
|
|
|
|
|
|
|
|
|
|
|
|
|
|
|
|
|
|
|
|
|
|
|
|
|
|
|
|
|
|
|
|
|
|
|
|
|
|
|
|
|
|
|
|
|
|
|
|
|
|
|
|
|
|
|
|
|
|
|
|
|
|
|
|
|
|
|
|
|
|
|
|
|
|
|
|
|
|
|
|
|
|
|
|
|
|
|
|
|
|
|
|
|
|
|
|
|
|
|
|
|
|
|
|
|
|
|
|
|
|
|
|
|
|
|
|
|
|
|
|
|
|
|
|
|
|
|
|
|
|
Fig. 20. Nonlinearity of intracellular viscoelastic response: two characteristic examples of viscoelastic response curves induced by force pulses of 200 pN and 50 pN, respectively. Note that the response induced by the large pulse is immediate but does not relax after switching o the pulse while for the small pulse the response is drastically delayed but is partially reversible.
The active force in Dictyostelia cells obtained in this way is about fact ≈ 30 pN which would correspond to the concerted action of seven kinesin motors. Active forces of the order of 30 pN are too weak to transport intracellular compartments such as mitochondria or vesicles through the cytoplasmic regions of high mechanical strength exhibiting typically yield forces of 300 pN. However, this problem is overcome by the viscoplastic behaviour of the cytoplasm. As demonstrated in Figure 20 the compartments can penetrate the high elasticity regions by extended application (for a few seconds) of forces considerably smaller then the yield force. After reaching the soft regions exhibiting yield strengths of 10 Pa rapid transport is possible again. The transport could be further accelerated by active softening of the intracellular regions of high mechanical strength mediated through intracellular signalling.
The situation is further obscured by the active adaptive response of the cells to mechanical stimuli and it is essential to develop further concepts and methods enabling the distinction between passive and active mechanical processes.

278 |
|
|
Physics of Bio-Molecules and Cells |
|||||
|
|
|
|
|
|
|
|
|
|
|
|
|
|
|
|
|
|
|
|
|
|
|
|
|
|
|
|
|
|
|
|
|
|
|
|
|
|
|
|
|
|
|
|
|
|
|
|
|
|
|
|
|
|
|
|
|
|
|
|
|
|
|
|
|
|
|
|
|
|
|
|
|
|
|
|
|
|
|
|
|
Fig. 21. a) Schematic view of micropipette aspiration technique. Note that aspiration occurs above a threshold pressure pth1. b) Phase contrast micrograph of Dictyostelia Discoideum cell aspired by a micropipette (inner diameter 3.8 µm) with constant suction pressure at the leading edge (left) and the trailing end of the cell (right). At the cell front, where a new pseudopod is forming, the aspiration length is significantly larger than at the trailing end of the cell. Black arrows denote the end of the aspirated cell lobe. Note that in both cases A and B the aspirated lobe exhibits a clear hyaline cap, free of internal cell compartments. The hyaline cap extends from the tip of the aspirated cell lobe to the beginning of the organelle-containing region (thin white arrow). The bold white arrow in B marks the pseudopod at the leading front of the cell.
7 Controll of cellular protrusions controlled by actin/myosin cortex
Another powerful micromechanical technique to study adaptive mechanical properties of cell envelopes and to gain insight into the coupling strength of the outer shell with the intracellular sca old is the micropipette aspiration technique (cf. Evans & Yeung 1989; Zhelev & Hochmuth 1995). An instructive example is shown in Figure 21. The cell envelope of Dictyostelia cells is aspirated by cylindrical pipettes with defined suction pressures (cf. Simson et al. 2000). Above a threshold pressure pth1 the cell envelope disrupts from the cell body and a tube-like protrusion moves into the pipette with nearly constant velocity until a finite protrusion length lc is reached (cf. Fig. 21a). After a recovery phase (typically 10–20 s) the protrusion is