
- •Analysis and Application of Analog Electronic Circuits to Biomedical Instrumentation
- •Dedication
- •Preface
- •Reader Background
- •Rationale
- •Description of the Chapters
- •Features
- •The Author
- •Table of Contents
- •1.1 Introduction
- •1.2 Sources of Endogenous Bioelectric Signals
- •1.3 Nerve Action Potentials
- •1.4 Muscle Action Potentials
- •1.4.1 Introduction
- •1.4.2 The Origin of EMGs
- •1.5 The Electrocardiogram
- •1.5.1 Introduction
- •1.6 Other Biopotentials
- •1.6.1 Introduction
- •1.6.2 EEGs
- •1.6.3 Other Body Surface Potentials
- •1.7 Discussion
- •1.8 Electrical Properties of Bioelectrodes
- •1.9 Exogenous Bioelectric Signals
- •1.10 Chapter Summary
- •2.1 Introduction
- •2.2.1 Introduction
- •2.2.4 Schottky Diodes
- •2.3.1 Introduction
- •2.4.1 Introduction
- •2.5.1 Introduction
- •2.5.5 Broadbanding Strategies
- •2.6 Photons, Photodiodes, Photoconductors, LEDs, and Laser Diodes
- •2.6.1 Introduction
- •2.6.2 PIN Photodiodes
- •2.6.3 Avalanche Photodiodes
- •2.6.4 Signal Conditioning Circuits for Photodiodes
- •2.6.5 Photoconductors
- •2.6.6 LEDs
- •2.6.7 Laser Diodes
- •2.7 Chapter Summary
- •Home Problems
- •3.1 Introduction
- •3.2 DA Circuit Architecture
- •3.4 CM and DM Gain of Simple DA Stages at High Frequencies
- •3.4.1 Introduction
- •3.5 Input Resistance of Simple Transistor DAs
- •3.7 How Op Amps Can Be Used To Make DAs for Medical Applications
- •3.7.1 Introduction
- •3.8 Chapter Summary
- •Home Problems
- •4.1 Introduction
- •4.3 Some Effects of Negative Voltage Feedback
- •4.3.1 Reduction of Output Resistance
- •4.3.2 Reduction of Total Harmonic Distortion
- •4.3.4 Decrease in Gain Sensitivity
- •4.4 Effects of Negative Current Feedback
- •4.5 Positive Voltage Feedback
- •4.5.1 Introduction
- •4.6 Chapter Summary
- •Home Problems
- •5.1 Introduction
- •5.2.1 Introduction
- •5.2.2 Bode Plots
- •5.5.1 Introduction
- •5.5.3 The Wien Bridge Oscillator
- •5.6 Chapter Summary
- •Home Problems
- •6.1 Ideal Op Amps
- •6.1.1 Introduction
- •6.1.2 Properties of Ideal OP Amps
- •6.1.3 Some Examples of OP Amp Circuits Analyzed Using IOAs
- •6.2 Practical Op Amps
- •6.2.1 Introduction
- •6.2.2 Functional Categories of Real Op Amps
- •6.3.1 The GBWP of an Inverting Summer
- •6.4.3 Limitations of CFOAs
- •6.5 Voltage Comparators
- •6.5.1 Introduction
- •6.5.2. Applications of Voltage Comparators
- •6.5.3 Discussion
- •6.6 Some Applications of Op Amps in Biomedicine
- •6.6.1 Introduction
- •6.6.2 Analog Integrators and Differentiators
- •6.7 Chapter Summary
- •Home Problems
- •7.1 Introduction
- •7.2 Types of Analog Active Filters
- •7.2.1 Introduction
- •7.2.3 Biquad Active Filters
- •7.2.4 Generalized Impedance Converter AFs
- •7.3 Electronically Tunable AFs
- •7.3.1 Introduction
- •7.3.3 Use of Digitally Controlled Potentiometers To Tune a Sallen and Key LPF
- •7.5 Chapter Summary
- •7.5.1 Active Filters
- •7.5.2 Choice of AF Components
- •Home Problems
- •8.1 Introduction
- •8.2 Instrumentation Amps
- •8.3 Medical Isolation Amps
- •8.3.1 Introduction
- •8.3.3 A Prototype Magnetic IsoA
- •8.4.1 Introduction
- •8.6 Chapter Summary
- •9.1 Introduction
- •9.2 Descriptors of Random Noise in Biomedical Measurement Systems
- •9.2.1 Introduction
- •9.2.2 The Probability Density Function
- •9.2.3 The Power Density Spectrum
- •9.2.4 Sources of Random Noise in Signal Conditioning Systems
- •9.2.4.1 Noise from Resistors
- •9.2.4.3 Noise in JFETs
- •9.2.4.4 Noise in BJTs
- •9.3 Propagation of Noise through LTI Filters
- •9.4.2 Spot Noise Factor and Figure
- •9.5.1 Introduction
- •9.6.1 Introduction
- •9.7 Effect of Feedback on Noise
- •9.7.1 Introduction
- •9.8.1 Introduction
- •9.8.2 Calculation of the Minimum Resolvable AC Input Voltage to a Noisy Op Amp
- •9.8.5.1 Introduction
- •9.8.5.2 Bridge Sensitivity Calculations
- •9.8.7.1 Introduction
- •9.8.7.2 Analysis of SNR Improvement by Averaging
- •9.8.7.3 Discussion
- •9.10.1 Introduction
- •9.11 Chapter Summary
- •Home Problems
- •10.1 Introduction
- •10.2 Aliasing and the Sampling Theorem
- •10.2.1 Introduction
- •10.2.2 The Sampling Theorem
- •10.3 Digital-to-Analog Converters (DACs)
- •10.3.1 Introduction
- •10.3.2 DAC Designs
- •10.3.3 Static and Dynamic Characteristics of DACs
- •10.4 Hold Circuits
- •10.5 Analog-to-Digital Converters (ADCs)
- •10.5.1 Introduction
- •10.5.2 The Tracking (Servo) ADC
- •10.5.3 The Successive Approximation ADC
- •10.5.4 Integrating Converters
- •10.5.5 Flash Converters
- •10.6 Quantization Noise
- •10.7 Chapter Summary
- •Home Problems
- •11.1 Introduction
- •11.2 Modulation of a Sinusoidal Carrier Viewed in the Frequency Domain
- •11.3 Implementation of AM
- •11.3.1 Introduction
- •11.3.2 Some Amplitude Modulation Circuits
- •11.4 Generation of Phase and Frequency Modulation
- •11.4.1 Introduction
- •11.4.3 Integral Pulse Frequency Modulation as a Means of Frequency Modulation
- •11.5 Demodulation of Modulated Sinusoidal Carriers
- •11.5.1 Introduction
- •11.5.2 Detection of AM
- •11.5.3 Detection of FM Signals
- •11.5.4 Demodulation of DSBSCM Signals
- •11.6 Modulation and Demodulation of Digital Carriers
- •11.6.1 Introduction
- •11.6.2 Delta Modulation
- •11.7 Chapter Summary
- •Home Problems
- •12.1 Introduction
- •12.2.1 Introduction
- •12.2.2 The Analog Multiplier/LPF PSR
- •12.2.3 The Switched Op Amp PSR
- •12.2.4 The Chopper PSR
- •12.2.5 The Balanced Diode Bridge PSR
- •12.3 Phase Detectors
- •12.3.1 Introduction
- •12.3.2 The Analog Multiplier Phase Detector
- •12.3.3 Digital Phase Detectors
- •12.4 Voltage and Current-Controlled Oscillators
- •12.4.1 Introduction
- •12.4.2 An Analog VCO
- •12.4.3 Switched Integrating Capacitor VCOs
- •12.4.6 Summary
- •12.5 Phase-Locked Loops
- •12.5.1 Introduction
- •12.5.2 PLL Components
- •12.5.3 PLL Applications in Biomedicine
- •12.5.4 Discussion
- •12.6 True RMS Converters
- •12.6.1 Introduction
- •12.6.2 True RMS Circuits
- •12.7 IC Thermometers
- •12.7.1 Introduction
- •12.7.2 IC Temperature Transducers
- •12.8 Instrumentation Systems
- •12.8.1 Introduction
- •12.8.5 Respiratory Acoustic Impedance Measurement System
- •12.9 Chapter Summary
- •References

Models for Semiconductor Devices Used in Analog Electronic Systems |
113 |
Pi hν
ID + IP
Vs l
E
w
d
Electrode
FIGURE 2.67
Geometry of a photoconductor slab.
2.6.5Photoconductors
Photoconductors (PCs) are transducers that convert photon energy into an increase in electrical conductance; they are also called light-dependent resistors or photoresistors. Figure 2.67 illustrates the cross-sectional schematic of a typical PC device. PCs can be made from a number of intrinsic and doped semiconductor materials. Each semiconductor has a distinct spectral response to light, ranging from UV to FIR. Table 2.1 lists some of the materials used in PCs, their bandgap energies in electronvolts, and the wavelength of their peak spectral response.
TABLE 2.1
Properties of Some Photoconductors
|
Bandgap |
Wavelength of Peak |
Rise Time/ |
PC Material |
Energy, eV |
Response, c, μm |
Fall Time |
|
|
|
|
ZnS |
3.60 |
0.345 |
|
CdS |
2.40 |
0.52 |
30 MS/10 MS |
CdSe |
1.80 |
0.69 |
15 MS/15 MS |
CdTe |
1.50 |
0.83 |
1 μs/1 μs |
Si (intrinsic) |
1.12 |
1.10 |
|
Ge (intrinsic) |
0.67 |
1.85 |
0.1 μs/0.1 μs |
PbS |
0.37 |
3.35 |
♠ 1 ns |
InAs |
0.35 |
3.54 |
|
Te |
0.33 |
7.75 |
|
PbTe |
0.30 |
4.13 |
2 μs |
PbSe |
0.27 |
4.58 |
|
HgCdTe (77 K) |
|
5.0 |
*/5 μs |
InSb (77 K) |
0.18 |
6.90 |
|
GeCu (4 K) |
|
25 |
|
GeBe (3 K) |
|
55 |
|
|
|
|
|
© 2004 by CRC Press LLC
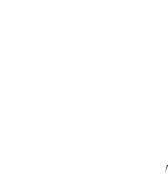
114 |
Analysis and Application of Analog Electronic Circuits |
In general, the total current in a PC can be written:
IPC = Vs [GD + GP] |
(2.205) |
where Vs is the bias voltage; GD is the equivalent dark conductance; and GP is the photoconductance. GP can be shown to be given by (Yang, 1988):
G = |
I |
P |
= |
q ητp (μp |
+ μn ) |
|
P |
λ |
˘ |
Siemens |
(2.206) |
|
|
|
|
|
i |
|
|||||||
|
|
|
2 |
|
|
|
|
˙ |
||||
P |
Vs |
|
l |
|
|
|
|
|
||||
|
|
|
|
|
hc ˚ |
|
|
where τp is the mean lifetime of holes; μp = vp/E = hole mobility in cm2/V.sec; μn = vn/E = electron mobility in cm2/V.sec; vp and vn are the mean drift velocities of holes and electrons, respectively; E is the uniform E-field in the semiconductor; and q, η, Pi, λ, h, and c have been defined previously. Note that, in general, μn > μp. The expression for GP is an approximation, valid up to the cut-off wavelength, λc. [Pi λ/hc] = Φi, the incoming number of photons/second on area wl m2. The dark conductance of a Si PC can be found simply from the room temperature resistivity of Si, ρ, and the geometry of the PC. For example:
GD = Aρl = wd
ρl = 0.2 cm ∞ 0.001 cm (2.3 ∞ 103 Ω cm ∞ 0.02 cm)
(2.207)
= 4.348 ∞ 10−6 S
From Equation 2.206, the photoconductance for a Si PC illuminated by 1 μW of 512-nm photons is:
|
Cb |
η |
τp |
cm2 V.sec |
W |
m |
|
˘ |
|
G = 1.6 ∞ 10−19 ∞ 0.8 |
∞ 10−4 |
(1350 + 450) |
10−6 ∞ 512 ∞ |
10−9 ˙ |
|||||
P |
|
10−4 |
|
|
6.625 ∞ 10−34 |
∞ 3 ∞ 103 |
˙ |
||
|
|
|
cm2 |
|
|
joule.sec |
|
m sec ˙ (2.208) |
|
|
|
|
|
|
|
|
|
˚ |
|
= 5.935 ∞ 10−4 |
Siemens |
|
|
|
|
|
|
A great advantage of PCs is their unique ability to respond to MIR and FIR photons at wavelengths not sensed by PIN PDs or APDs. For various materials, as the bandgap energy decreases, λc increases and the PC’s response time constants decrease.
PCs also are unique in their ability to convert x-ray photons to conductance change. X-ray sensing PCs are made of amorphous selenium (a-Se) (Soltani et al., 1999). An a-Se PC with E 10 V/μm produces approximately 1000 electron–hole pairs per 50-keV x-ray photon. There is a 50% attenuation of a 50-keV electron beam in d = 365 μm a-Se. Soltani et al. (1999) describe a charge-coupled x-ray photon-sensing array using a-Se sensors that have superior image resolution to phosphor x-ray sensors.
© 2004 by CRC Press LLC

Models for Semiconductor Devices Used in Analog Electronic Systems |
115 |
|
|
+5 V |
|
Pi |
|
|
|
RC |
|
−15 V |
ID RF |
|
|
|
|
IP + ID PC |
(0) |
|
Vo = IP RF
IOA
FIGURE 2.68
Op amp circuit for conditioning a photoconductive sensor’s output. The current through RC compensates for the PC’s dark current.
Figure 2.68 illustrates a simple op amp circuit that gives Vo Pi. Note that RC is used to cancel the PC’s dark current. A Wheatstone bridge can also be used to convert photoconductance to output voltage, albeit nonlinearly. Unlike PDs, PCs make thermal noise; it can be shown that the total MS noise current input to the op amp’s summing junction is:
|
= {4kT[GD + GP + GC + GF ]+ ina2 }B msA |
|
intot2 |
(2.209) |
Note that the MS noise increases with input light power because GP increases with Pi. In addition to the noise current, the op amp also has an equivalent short-circuit input noise voltage, ena2 B MSV. Chapter 9 will consider such noise.
2.6.6LEDs
Light-emitting diodes are widely used in all electronic applications as pilot lights, status indicators, and warning signals. They are also used in biomedical applications as (approximately) monochromatic light sources for chemical analysis by spectrophotometry. LEDs can be purchased that emit not only near IR, but also visible red, orange, yellow, green, blue, and white light. An important application for LEDs in biomedicine is the two light sources in the pulse oximeter, which is basically a two-wavelength spectrophotometer used to measure blood oxygen saturation (Northrop, 2002). One NIR LED emits at 805 nm, the isobestic wavelength for deoxyhemoglobin (Hb) and oxyhemoglobin (HbO2) absorbance (the wavelength at which Hb and HbO2 absorbances are equal). The other wavelength is at approximately 650 nm (red), where there is a large difference between the absorbances of Hb and HbO2. LEDs are also used as the light sources in the paper strip, blood glucose sensing systems. Again, a two-λ spectrophotometer is used. Even though LEDs are relative broadband emitters, their spectral purity is good enough for simple spectrophotometric measurements.
© 2004 by CRC Press LLC
116 |
Analysis and Application of Analog Electronic Circuits |
An LED is a solid-state, pn junction device that emits photons upon the application of a forward-biasing current. It converts electric energy directly into photon energy without the intermediate step of thermal conversion. LED p-material is typically doped gallium aluminum arsenide (GaAlAs), while the n-material is doped gallium arsenide (GaAs). Between the p and n layers is an active layer. When a forward voltage (and current) is applied to the LED, holes from the p-region (GaAlAs) meet electrons from the n-doped GaAs layer in the active layer and recombine, producing photons. Photon wavelength is dependent on the chemical composition and relative energy levels of the two doped semicon layers. It also depends to a small degree on the junction temperature and ID. Visible-light LEDs typically have plastic dome lenses that serve to expand and diffuse the light from the LED’s active layer. The plastic is colored to indicate the color of the emitted light; clear lenses are used for NIR LEDs.
The wavelength of the emitted light can be altered by varying the composition of the doped semicon materials used in the LED. Typical materials used in LED construction include Al, As, Ga, In, P, and N (as nitrides). White light can be made by several mechanisms, but one way is to make a blue LED and use the blue light to excite a mixture of phosphors in the reflector cup that emit at several longer wavelengths; the mixture of wavelengths appears white.
LED forward voltage is on the order of 1.5 V and operating currents range from a few to tens of milliamperes. When a forward current is applied through a pn junction, carriers are injected across the junction to establish excess carriers above the thermal equilibrium values. The excess carriers recombine and, in so doing, some energy is released in the form of heat and light (photons). The injected electrons in the p side make a downward energy transition from the conduction band to recombine with holes in the valence band. Photons are emitted having energy, Eg, in joules. The emission wavelength is approximately:
λ = hc/Eg meters |
(2.210) |
In practice, the emission power spectrum is not a narrow band such as that produced by lasers, but is a curve with a smooth peak and a Q defined by the wavelength of the peak emission power density divided by the Δλ
between the half-power wavelengths on either side of the peak. That is; Q = λpk/Δλ. For example, Q 16 for a GaAsP LED with peak emission at 650 nm
(Yang, 1988). The Qs for Osram LEDs LS5421, LO5411, LY5421, and LG5411 — emitting power peaks at 635, 610, 586 and 565 nm, respectively — are 14.1, 15.3, 13.0, and 22.6, respectively.
Figure 2.69(A) illustrates the I–V characteristics of a green gallium phosphide (GaP) LED. Compare this curve with the I–V curve for a typical small-signal, Si pn junction diode, as shown on Figure 2.69(B). Figure 2.69(C) illustrates the light intensity vs. ID for the green LED and Figure 2.70 illustrates the relative spectral emission of a GaP green LED. The peak is at approximately
© 2004 by CRC Press LLC