
- •Analysis and Application of Analog Electronic Circuits to Biomedical Instrumentation
- •Dedication
- •Preface
- •Reader Background
- •Rationale
- •Description of the Chapters
- •Features
- •The Author
- •Table of Contents
- •1.1 Introduction
- •1.2 Sources of Endogenous Bioelectric Signals
- •1.3 Nerve Action Potentials
- •1.4 Muscle Action Potentials
- •1.4.1 Introduction
- •1.4.2 The Origin of EMGs
- •1.5 The Electrocardiogram
- •1.5.1 Introduction
- •1.6 Other Biopotentials
- •1.6.1 Introduction
- •1.6.2 EEGs
- •1.6.3 Other Body Surface Potentials
- •1.7 Discussion
- •1.8 Electrical Properties of Bioelectrodes
- •1.9 Exogenous Bioelectric Signals
- •1.10 Chapter Summary
- •2.1 Introduction
- •2.2.1 Introduction
- •2.2.4 Schottky Diodes
- •2.3.1 Introduction
- •2.4.1 Introduction
- •2.5.1 Introduction
- •2.5.5 Broadbanding Strategies
- •2.6 Photons, Photodiodes, Photoconductors, LEDs, and Laser Diodes
- •2.6.1 Introduction
- •2.6.2 PIN Photodiodes
- •2.6.3 Avalanche Photodiodes
- •2.6.4 Signal Conditioning Circuits for Photodiodes
- •2.6.5 Photoconductors
- •2.6.6 LEDs
- •2.6.7 Laser Diodes
- •2.7 Chapter Summary
- •Home Problems
- •3.1 Introduction
- •3.2 DA Circuit Architecture
- •3.4 CM and DM Gain of Simple DA Stages at High Frequencies
- •3.4.1 Introduction
- •3.5 Input Resistance of Simple Transistor DAs
- •3.7 How Op Amps Can Be Used To Make DAs for Medical Applications
- •3.7.1 Introduction
- •3.8 Chapter Summary
- •Home Problems
- •4.1 Introduction
- •4.3 Some Effects of Negative Voltage Feedback
- •4.3.1 Reduction of Output Resistance
- •4.3.2 Reduction of Total Harmonic Distortion
- •4.3.4 Decrease in Gain Sensitivity
- •4.4 Effects of Negative Current Feedback
- •4.5 Positive Voltage Feedback
- •4.5.1 Introduction
- •4.6 Chapter Summary
- •Home Problems
- •5.1 Introduction
- •5.2.1 Introduction
- •5.2.2 Bode Plots
- •5.5.1 Introduction
- •5.5.3 The Wien Bridge Oscillator
- •5.6 Chapter Summary
- •Home Problems
- •6.1 Ideal Op Amps
- •6.1.1 Introduction
- •6.1.2 Properties of Ideal OP Amps
- •6.1.3 Some Examples of OP Amp Circuits Analyzed Using IOAs
- •6.2 Practical Op Amps
- •6.2.1 Introduction
- •6.2.2 Functional Categories of Real Op Amps
- •6.3.1 The GBWP of an Inverting Summer
- •6.4.3 Limitations of CFOAs
- •6.5 Voltage Comparators
- •6.5.1 Introduction
- •6.5.2. Applications of Voltage Comparators
- •6.5.3 Discussion
- •6.6 Some Applications of Op Amps in Biomedicine
- •6.6.1 Introduction
- •6.6.2 Analog Integrators and Differentiators
- •6.7 Chapter Summary
- •Home Problems
- •7.1 Introduction
- •7.2 Types of Analog Active Filters
- •7.2.1 Introduction
- •7.2.3 Biquad Active Filters
- •7.2.4 Generalized Impedance Converter AFs
- •7.3 Electronically Tunable AFs
- •7.3.1 Introduction
- •7.3.3 Use of Digitally Controlled Potentiometers To Tune a Sallen and Key LPF
- •7.5 Chapter Summary
- •7.5.1 Active Filters
- •7.5.2 Choice of AF Components
- •Home Problems
- •8.1 Introduction
- •8.2 Instrumentation Amps
- •8.3 Medical Isolation Amps
- •8.3.1 Introduction
- •8.3.3 A Prototype Magnetic IsoA
- •8.4.1 Introduction
- •8.6 Chapter Summary
- •9.1 Introduction
- •9.2 Descriptors of Random Noise in Biomedical Measurement Systems
- •9.2.1 Introduction
- •9.2.2 The Probability Density Function
- •9.2.3 The Power Density Spectrum
- •9.2.4 Sources of Random Noise in Signal Conditioning Systems
- •9.2.4.1 Noise from Resistors
- •9.2.4.3 Noise in JFETs
- •9.2.4.4 Noise in BJTs
- •9.3 Propagation of Noise through LTI Filters
- •9.4.2 Spot Noise Factor and Figure
- •9.5.1 Introduction
- •9.6.1 Introduction
- •9.7 Effect of Feedback on Noise
- •9.7.1 Introduction
- •9.8.1 Introduction
- •9.8.2 Calculation of the Minimum Resolvable AC Input Voltage to a Noisy Op Amp
- •9.8.5.1 Introduction
- •9.8.5.2 Bridge Sensitivity Calculations
- •9.8.7.1 Introduction
- •9.8.7.2 Analysis of SNR Improvement by Averaging
- •9.8.7.3 Discussion
- •9.10.1 Introduction
- •9.11 Chapter Summary
- •Home Problems
- •10.1 Introduction
- •10.2 Aliasing and the Sampling Theorem
- •10.2.1 Introduction
- •10.2.2 The Sampling Theorem
- •10.3 Digital-to-Analog Converters (DACs)
- •10.3.1 Introduction
- •10.3.2 DAC Designs
- •10.3.3 Static and Dynamic Characteristics of DACs
- •10.4 Hold Circuits
- •10.5 Analog-to-Digital Converters (ADCs)
- •10.5.1 Introduction
- •10.5.2 The Tracking (Servo) ADC
- •10.5.3 The Successive Approximation ADC
- •10.5.4 Integrating Converters
- •10.5.5 Flash Converters
- •10.6 Quantization Noise
- •10.7 Chapter Summary
- •Home Problems
- •11.1 Introduction
- •11.2 Modulation of a Sinusoidal Carrier Viewed in the Frequency Domain
- •11.3 Implementation of AM
- •11.3.1 Introduction
- •11.3.2 Some Amplitude Modulation Circuits
- •11.4 Generation of Phase and Frequency Modulation
- •11.4.1 Introduction
- •11.4.3 Integral Pulse Frequency Modulation as a Means of Frequency Modulation
- •11.5 Demodulation of Modulated Sinusoidal Carriers
- •11.5.1 Introduction
- •11.5.2 Detection of AM
- •11.5.3 Detection of FM Signals
- •11.5.4 Demodulation of DSBSCM Signals
- •11.6 Modulation and Demodulation of Digital Carriers
- •11.6.1 Introduction
- •11.6.2 Delta Modulation
- •11.7 Chapter Summary
- •Home Problems
- •12.1 Introduction
- •12.2.1 Introduction
- •12.2.2 The Analog Multiplier/LPF PSR
- •12.2.3 The Switched Op Amp PSR
- •12.2.4 The Chopper PSR
- •12.2.5 The Balanced Diode Bridge PSR
- •12.3 Phase Detectors
- •12.3.1 Introduction
- •12.3.2 The Analog Multiplier Phase Detector
- •12.3.3 Digital Phase Detectors
- •12.4 Voltage and Current-Controlled Oscillators
- •12.4.1 Introduction
- •12.4.2 An Analog VCO
- •12.4.3 Switched Integrating Capacitor VCOs
- •12.4.6 Summary
- •12.5 Phase-Locked Loops
- •12.5.1 Introduction
- •12.5.2 PLL Components
- •12.5.3 PLL Applications in Biomedicine
- •12.5.4 Discussion
- •12.6 True RMS Converters
- •12.6.1 Introduction
- •12.6.2 True RMS Circuits
- •12.7 IC Thermometers
- •12.7.1 Introduction
- •12.7.2 IC Temperature Transducers
- •12.8 Instrumentation Systems
- •12.8.1 Introduction
- •12.8.5 Respiratory Acoustic Impedance Measurement System
- •12.9 Chapter Summary
- •References
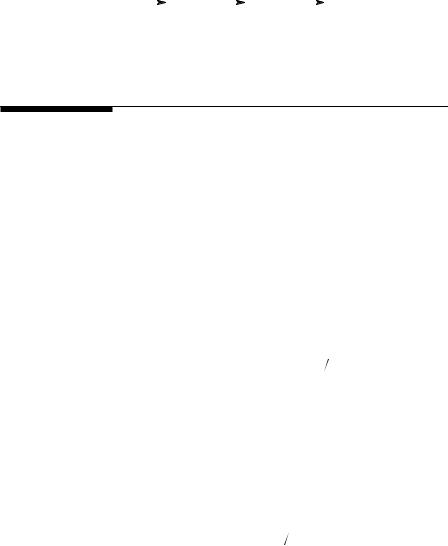
346 |
|
Analysis and Application of Analog Electronic Circuits |
|||||
|
Si(f) |
|
|
|
|
So(f) |
|
|
|
H(jf) |
|
G(jf) |
|||
|
|
|
|
|
|
||
|
|
|
|
|
|||
|
|
|
|
|
|
|
|
FIGURE 9.8
Two cascaded linear systems through which Gaussian noise is propagating.
9.3Propagation of Noise through LTI Filters
In a formal rigorous treatment of noise in linear systems, it is possible to show that the PDF of the output of a linear system is Gaussian, given a Gaussian noise input. In addition, it can be shown rigorously (James et al., 1947) that the PDS of the system’s output noise is given by
Sy (f ) = Sx (f ) |
|
H(j 2πf ) |
|
2 |
MS units Hz |
(9.41) |
|
|
This is the scalar product of the positive-real input PDS and the magnitude squared of the LTI system’s transfer function. This relation can be extended to include two or more cascaded systems, as shown in Figure 9.8.
Sy (f ) = Sx (f ) |
|
H(j 2π f ) |
|
2 |
|
G(j 2π f ) |
|
2 |
MSV Hz |
(9.42) |
|
|
|
|
or
Sy (f ) = Sx (f ) |
|
H(j 2π f )G(j 2π f ) |
|
2 |
MSV Hz |
(9.43) |
|
|
If white noise with a PDS, Sx(f ) = η MSV/Hz is the input to a linear system, then the output PDS is simply:
|
|
Sy (f ) = η |
|
H(j 2π f ) |
|
2 |
MSV Hz |
(9.44) |
||||
|
|
|||||||||||
The total mean-squared output noise of this system is given by: |
|
|||||||||||
|
|
= • Sy (f )df = η • |
|
H(j 2π f ) |
|
2 df MSV |
|
|||||
|
von2 |
(9.45) |
||||||||||
|
|
|
||||||||||
|
|
|
|
|
||||||||
0 |
0 |
|
|
|
|
|
|
|
|
For transfer functions with one more finite poles than zeros, the right-hand integral of Equation 9.45 may be shown to be the product of the transfer function’s low-frequency or mid-band gain squared times the filter’s equivalent
© 2004 by CRC Press LLC

Noise and the Design of Low-Noise Amplifiers for Biomedical Applications |
347 |
||||
|
TABLE 9.1 |
|
|
|
|
|
Gain2-Bandwidth Products for Some Common Filter Transfer Functions |
|
|
||
|
Transfer Functiona |
Gain2 Bandwidthb |
Filter Type |
|
|
1. |
|
Kv |
|
|
|
|
|
|
||
sτ + 1 |
|
|
|
|
||||||
|
|
|
|
|
||||||
2. |
|
|
|
|
Kv |
|
|
|
||
( |
sτ |
1 |
+ 1 |
sτ |
2 |
+ 1 |
|
|||
|
|
)( |
|
) |
|
|||||
3. |
|
|
|
|
Kv |
|
|
|
||
s2 |
ωn2 + s2ξ ωn + 1 |
|||||||||
|
||||||||||
4. |
|
|
sKv (2ξ ωn ) |
|||||||
s2 |
ωn2 + s2ξ ωn + 1 |
|||||||||
|
2 |
|
1 ˆ |
|
|
|
Kv |
|
|
˜ |
|
Low pass; one real-pole |
|
|
4τ ↓ |
|
|
|
2 |
|
|
1 |
ˆ |
Low pass; two real poles |
Kv |
4(τ1 + τ2 ) |
↓˜ |
|||
2 |
|
ωn |
ˆ |
|
Low pass; underdamped quadratic |
Kv |
|
8ξ |
˜ |
|
|
|
|
↓ |
|
|
|
Kv2 (ξωn ) |
|
Underdamped quadratic band pass |
5. |
|
|
|
sτ1Kv |
|
|
2 |
|
1 |
|
ˆ |
Overdamped quadratic band pass |
|
|
|
|
|
|
|
|
Kv |
|
|
|
˜ |
||
( |
sτ |
|
+ 1 |
sτ |
|
+ 1 |
|
||||||
|
1 |
2 |
|
4τ2 (1+ τ2 |
τ1 )↓ |
|
|||||||
|
|
)( |
|
) |
|
|
aH(s)
bHz
Hertz noise bandwidth. Thus, the filter’s gain-squared bandwidth product is given by:
GAIN2BW = • |
|
H(2π fj) |
|
2 df |
(9.46) |
|
|
||||
|
|
|
|||
0 |
|
|
|
|
|
Gain2-bandwidth integrals have been evaluated for a number of transfer functions using complex variable theory (James et al., 1947). Table 9.1 gives the gain2-bandwidth integrals for five common transfer functions. Note that the equivalent noise bandwidths (in brackets in each case) are in Hertz, not radians/second. Also note the absence of any 2π factors in these expressions. Gain2-bandwidth integrals are used to estimate the total MS output noise from amplifiers with (approximate) white noise input sources and are thus useful in calculating output signal-to-noise ratios.
9.4Noise Factor and Figure of Amplifiers
9.4.1Broadband Noise Factor and Noise Figure of Amplifiers
An amplifier’s noise factor, F, is defined as the ratio of the mean-squared signal-to-noise ratio at the amplifier’s input to the MS signal-to-noise ratio at the amplifier’s output. Because a real amplifier is noisy and adds noise to the signal as well as amplifying it, the output signal-to-noise ratio (SNRo)
© 2004 by CRC Press LLC

348 |
Analysis and Application of Analog Electronic Circuits |
|
|
ena |
Vi |
|
Rs @ T |
|
|
|
Vo |
|
|
+ |
Vs |
ina |
Vi H(jf) |
Amplifier
FIGURE 9.9
The simple two-noise source model for a noisy VCVS.
is always less than the input signal-to-noise ratio (SNRi); thus, the noise factor is always greater than one for a noisy amplifier. F is a figure of merit for an amplifier — the closer to unity the better.
F ∫ |
SNRi |
> 1 |
(9.47) |
|
|||
|
SNRo |
|
|
The noise figure is defined as: |
|
||
NF ∫ 10 log10 (F) (decibels dB) |
(9.48) |
when the SNRs are in terms of mean squared quantities. The closer NF is to zero, the quieter the amplifier is.
Figure 9.9 illustrates a simple two-noise source model for a noisy amplifier. Here it is assumed the spectrums of ena and ina are white and that R1 Rs.
The MS input signal is Si = vs2 , so the MS output signal is So = KV2 vs2 , where KV2 is the amplifier’s mid-band gain squared. The MS input noise is simply that associated with vs (here set to zero) plus the Johnson noise from the source resistance, Rs, in a specified Hertz noise bandwidth, B. It is:
Ni = 4kTRs B MSV |
(9.49) |
The mean-squared noise at the amplifier’s output, No, is composed of three components: one from the Rs Johnson noise and two from the equivalent noise sources. No can be written as the sum of MS voltages:
No = (4kTRs + ena2 |
+ ina2 |
Rs2 )• |
|
H(j 2π f ) |
|
2 df |
|
|
|||||
|
|
(9.50) |
||||
|
|
0 |
||||
= (4kTRs + ena2 |
+ ina2 |
Rs2 )Kv2 B MSV |
Using the definition for F, the noise factor for the simple noisy amplifier model can be written as:
© 2004 by CRC Press LLC
Noise and the Design of Low-Noise Amplifiers for Biomedical Applications |
349 |
||||
F = 1+ |
e 2 |
+ i 2 |
R 2 |
(9.51) |
|
na |
na |
s |
|
||
|
|
|
4kTRs
Note that this expression for F contains no bandwidth terms; they cancel out. When the NF is given for an amplifier, Rs must be specified, as well as the Hertz bandwidth, B, over which the noise is measured. The temperature should also be specified, although common practice usually sets T at 298 K (25∞C).
For practical amplifiers, NF and F are functions of frequency because ena and ina are functions of frequency (see Figure 9.6). For a given Rs, F tends to rise at low frequencies due to the 1/f components in the equivalent input noise sources. F also increases at high frequencies — again, due to the high-fre- quency increases in ena and ina. Often, one is interested in the noise performance of an amplifier in low or high frequencies in which the NF and F are not minimum. To examine the detailed noise performance of an amplifier at low and high frequencies, use the spot noise figure (described next).
9.4.2Spot Noise Factor and Figure
Spot noise measurements are made through a narrow band-pass filter in order to evaluate an amplifier’s noise performance in a certain narrow frequency range, particularly where ena(f ) and ina(f ) are not constant, such as the 1/f range. Figure 9.10 illustrates a set of spot noise figure (SNF) contours for a commercial low-noise preamplifier with applications at audio frequencies. Note the area in {Rs, f } space at which the spot noise figure is a minimum. For best noise performance, the Thevenin resistance of the source, Rs, should lie in the range of minimum SNF and the input signal’s PDS should contain most of its energy in the range of frequencies at which the SNF is minimum.
A system for determining an amplifier’s spot noise figure is shown in Figure 9.11. An adjustable white noise voltage source is used at the amplifier’s input. Note that the output resistance of the white noise source plus some external resistance must add up to Rs, the specified Thevenin equivalent input resistance. The system is used as follows: first, the band-pass filter (BPF) is set to the desired center frequency, fc, around which the amplifier’s noise performance is to be characterized. Then the white noise generator is set to eN = 0. Assume that the total mean squared noise at the system output under these conditions can be written as:
No (fc ) = [4kTRs + ena2 (fc )+ ina2 (fc )Rs2 ] KV2 BF MSV |
(9.52) |
where ena(fc) is the value of ena at the center frequency; fc, BF is the equivalent noise bandwidth of the BPF; and KV is the combined gain of the amplifier under measurement at fc, the BPF at fc, and the postamplifier. KV can be written:
© 2004 by CRC Press LLC

350 |
|
|
|
|
Analysis and Application of Analog Electronic Circuits |
||||||||||||
RS (ohms) |
|
|
|
|
|
|
|
|
|
|
|
|
|
|
|
||
109 |
|
|
|
|
|
|
|
|
|
|
|
|
|
|
|
|
|
|
|
|
|
|
|
|
|
|
10 dB |
|
|
|
|
|
20 dB |
|
|
|
|
|
|
|
|
|
|
|
|
|
|
|
|
|
|
||
|
|
|
|
|
|
|
|
|
|
|
|
|
|
|
|
|
|
108 |
|
|
|
|
|
|
|
|
|
|
|
|
|
|
|
|
|
|
|
|
|
|
|
|
|
|
1 dB |
|
|
|
|
|
|
|
|
|
|
|
|
|
|
|
|
|
|
|
|
|
|
|
|
|
|
107 |
|
|
|
|
|
|
|
|
|
|
|
|
|
|
|
|
|
|
|
|
|
|
|
|
|
|
0.1 dB |
|
|
|
|
|
|
|
|
|
|
|
|
|
|
|
|
|
|
|
|
|
|
|
|
|
|
106 |
|
|
|
|
|
|
|
|
|
|
|
|
|
|
|
|
|
|
|
|
|
|
|
|
|
< 0.05 dB |
|
|
|
|
|
|
|||
|
|
|
|
|
|
|
|
|
|
|
|
|
|
|
|||
105 |
|
|
|
|
|
|
|
|
|
|
|
|
|
|
|
|
|
|
|
|
|
|
10 dB |
|
|
|
|
|
|
|
|
|
|
|
|
|
|
|
|
|
|
|
|
|
|
|
|
|
|
|
|
|
|
104 |
|
|
|
|
|
|
|
|
|
|
|
|
|
|
|
|
|
|
|
|
|
|
|
|
|
|
|
|
|
1 dB |
|
|
|
|
|
|
|
|
|
|
|
|
|
|
|
|
|
|
|
|
|
|
|
103 |
|
|
|
|
|
20 dB |
|
|
|
|
|
|
|
|
|
|
|
|
|
|
|
|
|
|
|
|
|
|
|
|
|
|
|
|
|
|
|
|
|
|
30 dB |
|
|
|
|
|
|
|
|
|
|
|
|
102 |
|
|
|
|
|
|
|
|
|
|
|
|
|
|
|
|
|
|
|
|
|
|
|
|
|
|
|
|
|
|
|
|
|
|
|
|
|
|
|
|
40 dB |
|
|
|
|
|
|
|
|
|
|
|
|
|
|
|
|
|
|
|
|
|
|
|
|
|
|
|
|
|
|
10 |
|
|
|
|
|
|
|
|
|
|
|
|
|
|
|
|
|
|
|
|
|
|
|
|
|
|
|
|
|
|
|
|
|
|
|
|
10−2 |
10−1 |
1 |
10 |
102 |
103 |
104 |
105 |
106 |
||||||||
|
|
|
|
|
|
|
f |
(Hz) |
|
|
|
|
|
|
FIGURE 9.10
Curves of constant spot noise figure (SNF) for a typical commercial low-noise amplifier. Note the region in RS-f space at which the SNF is minimum (optimum).
Rs @ T |
ena |
Vi |
Von |
|
|||
|
|
|
+ |
eN |
ina |
|
Vi H(j2πf) |
|
|
|
Ideal BPF |
|
|
|
Vof |
KF |
|
|
|
|
BF |
0 |
|
|
0 |
fc |
f |
KAVof
KA
True rms
Differential
voltmeter
amplifier
FIGURE 9.11
A test circuit for measuring an amplifier’s SNF.
© 2004 by CRC Press LLC
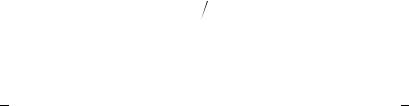
Noise and the Design of Low-Noise Amplifiers for Biomedical Applications |
351 |
KV = H(j 2π fc) KF KA |
(9.53) |
In the second step, the white noise source is made nonzero and adjusted so that the true RMS meter reads 2 higher than in the first case with eN = 0. The MS output voltage can now be written:
No′ (fc ) = 2No (fc ) = 2[4kTRs + ena2 (fc )+ ina2 (fc )Rs2 ]Kv2BF
= [ |
|
|
+ 4kTRs + ena2 (fc )+ ina2 (fc )Rs2 ]Kv2 BF |
(9.54) |
|||
eN2 |
|
||||||
Under this condition, it is evident that |
|
||||||
|
|
|
= 4kTRs + ena2 (fc )+ ina2 (fc )Rs2 |
|
|||
|
eN2 |
(9.55) |
|||||
so: |
|
||||||
[ena2 (fc )+ ina2 (fc )Rs2 ]= [ |
|
− 4kTRs ] |
|
||||
eN2 |
(9.56) |
If the left-hand side of this equation is substituted into Equation 9.51 for the noise factor, F,
Fspot = |
|
(4kTRs ) |
|
eN2 |
(9.57) |
Note that this simple expression for the SNF does not contain specific terms for the band-pass filter’s center frequency, fc, or its Hertz noise band-
width, BF. Note that these parameters must be specified when giving Fspot for an amplifier. Fspot is actually calculated by setting fc and Rs, then determining
the eN2 value that doubles the mean squared output noise. This value of eN2 is then divided by the calculated white noise spectrum from the resistor Rs.
It is also possible to measure Fspot using a sinusoidal source of frequency fc, instead of the calibrated white noise source, eN. See the home problems at the end of this chapter for a detailed treatment of this method.
9.4.3Transformer Optimization of Amplifier NF and Output SNR
Figure 9.10 illustrates that, for a given set of internal biasing conditions, a given amplifier will have an optimum operating region in which NFspot is a minimum in Rs,fc-space. In some practical instances, the input transducer to which the amplifier is connected has an Rs far smaller than the Rs giving the lowest NFspot on the amplifier’s spot NF contours. Consequently, the signal conditioning system (i.e., transducer and amplifier) is not operating to give the lowest NF or the highest output SNR.
© 2004 by CRC Press LLC

352 |
Analysis and Application of Analog Electronic Circuits |
|||
RS @ T |
1 : n |
|
ena |
Vo |
|
|
|||
|
|
|
|
Vi |
+ |
R1 |
ina |
|
+ |
VS |
|
Vi H(jω) |
||
|
|
|
Ideal Xfmr.
FIGURE 9.12
Use of an ideal transformer in an amplifier’s input circuit to maximize the output MS signal- to-noise ratio, as well as minimize the SNF, given the optimum turns ratio.
One way of improving the output SNR is to couple the input transducer to the amplifier through a low-noise, low-loss transformer, as shown in Figure 9.12. Such coupling, of course, presumes that the signal coming from the transducer is ac and not dc, for obvious reasons. (A practical transformer is a band-pass device that loses efficiency at low and high frequencies, thus limiting the range of frequencies over which output SNR can be maximized.)
The output MS SNR can be calculated for the circuit of Figure 9.12 as follows: the MS input signal is simply vs2 . In the case of a sinusoidal input, it is well known that vs2 = Vs2/2 MSV, where Vs is the peak value of the sinusoid. The MS signal at the output is:
|
|
|
|
|
S = v 2 n2 |
K 2 |
(9.58) |
||
o s |
v |
|
where n is the transformer’s secondary-to-primary turns ratio and KV is the amplifier’s mid-band gain.
The transformer is assumed to be ideal (and noiseless). In practice, transformer windings have finite resistance and thus make Johnson noise; their magnetic cores contribute Barkhausen noise to their outputs. (Barkhausen noise arises from the small transient voltages induced on the transformer winding when magnetic domains in the transformer’s ferromagnetic core flip direction as the magnetizing field, H, varies in time. Domain flipping is effectively random at low H values.)
The ideal transformer, besides having infinite frequency response, is also lossless and noiseless. From this latter assumption, it is easy to show that the amplifier “sees” a transformed Thevenin equivalent circuit of the input transducer with an open-circuit voltage of n vs(t) and a Thevenin resistance of n2 Rs (Northrop, 1990). Thus, the mean squared output noise of the trans- former-input amplifier can be written
No |
|
2 |
2 |
2 2 |
2 |
˘ |
2 |
B MSV |
(9.59) |
= n |
|
4kTRs + ena |
+ ina (n |
Rs ) |
˙ Kv |
||||
|
|
|
|
|
|
˚ |
|
|
|
© 2004 by CRC Press LLC