
Principles and Applications of Asymmetric Synthesis
.pdf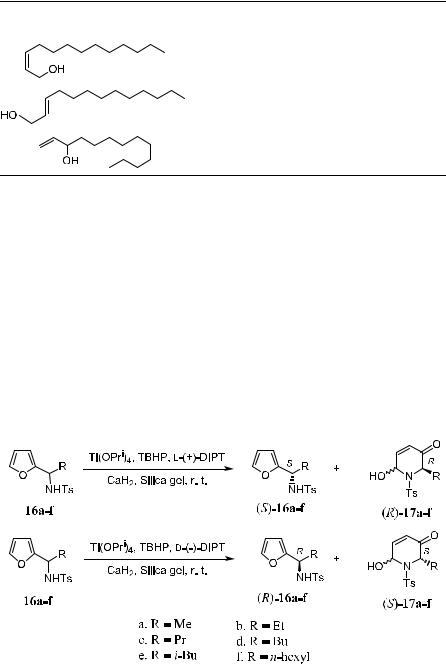
4.1 ASYMMETRIC EPOXIDATION OF ALLYLIC ALCOHOLS: SHARPLESS EPOXIDATION |
201 |
TABLE 4±1. Asymmetric Epoxidation of Allylic Alcohols by Sharpless Reagents (Method A) and by the Modi®ed Sharpless Reagents (Method B)
|
|
Time |
Yield |
|
ee |
|
Substrate |
Method |
(h) |
(%) |
[a]D |
(%) |
Con®g. |
|
A |
96 |
76±80 |
ÿ7.6 |
95 |
2R,3S |
|
B |
8 |
76 |
ÿ7.8 |
|
|
|
A |
72 |
76±80 |
‡26.5 |
96 |
2S,3S |
|
B |
6 |
76.4 |
‡25.9 |
|
|
|
A |
360 |
81 |
‡16.2 |
91 |
2S,3S |
|
B |
25 |
84 |
‡15.2 |
|
|
Conditions: Method A: Epoxidation using Sharpless reagent; method B: addition of 0.05±0.1 equivalent of calcium hydride and 0.1±0.15 equivalent of silica gel to the Sharpless reagent.
ee ˆ Enantiomeric excess.
slowly. For example, no reaction occurs when 0.6 equivalent of TBHP is used. This reaction is important because the furan ring compounds (S)-16a±f can be converted to a-amino acids by ozonolysis or oxidation using RuCl3/NaIO4.
Zhou and Wei14 then further extended the modi®ed epoxidation to kinetic resolution of a-pyrroyl carbinol (Scheme 4±8).
The reaction was carried out in CH2Cl2 using Ti(OPri)4 (1.0 equivalent), l- (‡)-DIPT or d-(ÿ)-DIPT (1.2 equivalents), 5±10 mol% of CaH2, 10±20% of silica gel, and TBHP (1.0 equivalent) at ÿ10 C. The substituent R can be a primary or secondary alkyl group. The presence of a tosyl substituent on the
Scheme 4±7
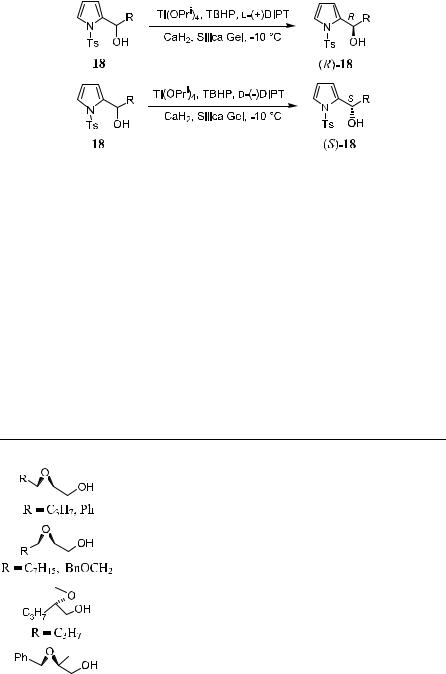
202 ASYMMETRIC OXIDATIONS
Scheme 4±8
nitrogen atom avoids N-oxide formation, and kinetic resolution takes place with high enantioselectivity (90±95% ee). When l-(‡)-DIPT is used, the slow reacting enantiomer is the one related to (R)-18, while (S)-18 remains as the slow reacting enantiomer when d-(ÿ)-DIPT is employed (Scheme 4±8).
4.1.3.2The 4 AÊ Molecular Sieves System. The initial procedure for the
Sharpless reaction required a stoichiometric amount of the tartrate±Ti complex
Ê
promoter. In the presence of 4 A molecular sieves, the asymmetric reaction can be achieved with a catalytic amount of titanium tetraisopropoxide and DET (Table 4±2).15 This can be explained by the fact that the molecular sieves may remove the co-existing water in the reaction system and thus avoid catalyst deactivation. Similar results may be observed in kinetic resolution (Table 4±3).15
TABLE 4±2. Catalytic Asymmetric Epoxidation With (B)-DET
Product |
|
|
|
|
Ti±Tartrate |
Temp. ( C) |
Time (h) |
Yield (%) |
ee (%) |
|||
|
|
|
|
5/6.0 |
ÿ20 |
2.5 |
85 |
94 |
||||
|
|
|
|
5/7.5 |
ÿ20 |
3 |
89 |
>98 |
||||
|
|
|
|
10/14 |
ÿ10 |
29 |
74 |
86 |
||||
|
|
|
|
|
10/14 |
ÿ20 |
43 |
|
85 |
|||
|
|
|
|
|
|
4.7/5.9 |
ÿ12 |
11 |
88 |
95 |
||
|
|
|
|
|
||||||||
|
|
|
|
5/7.5 |
ÿ35 |
2 |
79 |
>98 |
||||
|
|
|
|
|
|
|
|
|
|
|
|
|
ee ˆ Enantiomeric excess.
Reprinted with permission by Am. Chem. Soc., Ref. 15.
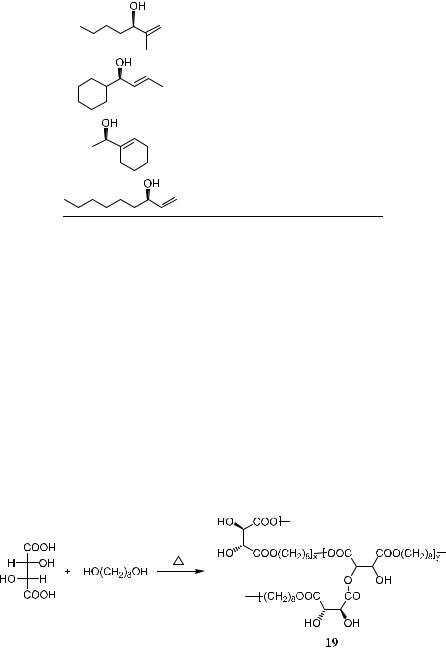
4.1 ASYMMETRIC EPOXIDATION OF ALLYLIC ALCOHOLS: SHARPLESS EPOXIDATION |
203 |
|||||
|
TABLE 4±3. Kinetic Resolution with (B)-DIPT |
|
|
|
||
|
|
|
|
|
|
|
|
Product |
Yield (%) |
Conversion (%) |
ee (%) |
|
|
|
|
|
|
|
|
|
|
|
93 |
53 |
94 |
|
|
|
|
96 |
54 |
94 |
|
|
|
|
93 |
63 |
>98 |
|
|
|
|
92 |
51 |
86 |
|
|
ee ˆ Enantiomeric excess.
Reprinted with permission by Am. Chem. Soc., Ref. 15.
4.1.3.3 Asymmetric Epoxidation Using Polymer-Supported Ti(IV) Catalysts. The advantages of polymer-supported reactive species are now widely recognized by organic chemists. The strategy often a¨ords several advantages over the use of homogeneous catalysts. One advantage of these polymersupported catalysts is their ease of separation from the reaction system, which allows their e½cient recovery and potential reuse. Using polymer-supported catalysts also makes it possible to carry out the reactions in ¯ow reactors or ¯ow membrane reactors for continuous production.16
Canali et al.17 reported the use a linear poly(tartrate) ligand in the asymmetric epoxidation of allylic alcohols. Moderate results were obtained. They also reported the use of branched/crosslinked poly(tartrate), which gave moderate to good results in the asymmetric epoxidation of allylic alcohols. As shown in Scheme 4±9, when l-(‡)-tartaric acid and 1,8-octanediol are heated
Scheme 4±9
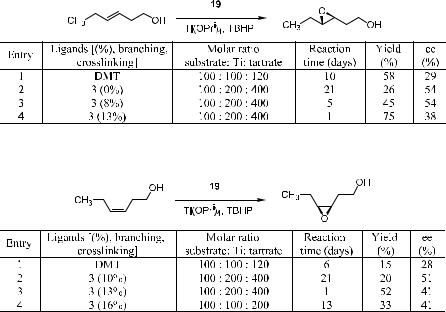
204 ASYMMETRIC OXIDATIONS
Scheme 4±10
Scheme 4±11
together, condensation polymerization takes place, yielding the branched/ crosslinked polyester 19, which shows moderate to high asymmetric induction capability for the asymmetric epoxidation of allylic alcohols.18
As discussed thus far, Sharpless epoxidation deals with allylic alcohol substrates, giving 2,3-epoxy alcohols as the reaction product. 3,4-Epoxy alcohols are also important building blocks in organic synthesis. However, only a few reports about the enantioselective synthesis of 3,4-epoxy alcohols have been published,19 and the results were not as satisfactory as those with 2,3-epoxy alcohols. Karjalainen et al.20 found that, in the presence of the branched/ crosslinked poly(tartrate), epoxidation of 3-en-1-ol substrates proceeded readily, producing the corresponding 3,4-epoxy alcohols with moderate yields but higher enantiomeric excess as compared with that obtained from a reaction catalyzed by monomeric tartaric acid esters. As shown in Schemes 4±10 and 4± 11, polymer catalyst 19 facilitates the asymmetric epoxidation of 3-en-1-ol compounds, providing product with much higher ee than that from monomer DMT (dimethyl tartrate)±mediated reactions.
4.2SELECTIVE OPENING OF 2,3-EPOXY ALCOHOLS
Sharpless epoxidation is considered highly valuable because it combines the powerful nature of the reaction with the capacity of the resultant epoxy alco-
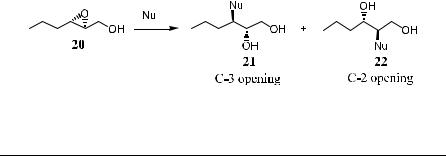
4.2 SELECTIVE OPENING OF 2,3-EPOXY ALCOHOLS |
205 |
hols to undergo regioselective and stereoselective reactions with various nucleophiles. The regiochemistry is often determined by the functional group in the substrate or by chelation between substrates and the reagents.
4.2.1External Nucleophilic Opening of 2,3-Epoxy Alcohols
4.2.1.1 Ti(OPri)4-Mediated Nucleophilic Opening of 2,3-Epoxy Alcohols. Caron and Sharpless21 have demonstrated that in the presence of 1.5 equivalents of Ti(OPri)ÿ4 1, nucleophiles such as secondary amine, azide, thiol, and free alcohol preferentially attack the C-3 atom in chiral 2,3-epoxy alcohols with con®guration inversion at the C-3 position. Excess nucleophile can be used to overcome the regiochemical problems associated with the inherently low reaction rate. In the absence of Ti(IV) compounds, ring opening does not occur (Table 4±4). For a wide variety of nucleophiles, Ti(OPri)4 not only enhances the rate of nucleophilic ring opening of 2,3-epoxy alcohols, but also leads to an increase in regioselectivity for C-3 attack in the reaction.
Ti(OPri)4-mediated nucleophilic ring opening of 2,3-epoxy-alcohol with primary amine requires more rigorous conditions, and the product is a complex mixture. Lin and Zeng22 found that this problem could be overcome and moderate to good yields could be obtained under weak base conditions by in situ N-acylation of the aminolysis product with benzoyl chloride.
TABLE 4±4. Nucleophilic Opening of 3-Propyloxiranemethanol21
|
Ti(OPri)4 |
|
Regioselectivity |
Yield |
Nucleophile |
(eq.) |
Reaction Conditions |
(C-3/C-2) |
(%) |
|
|
|
|
|
Et2NH |
0 |
Et2NH (excess), re¯ux, 18 h |
3.7/1 |
4 |
Et2NH |
1.5 |
Et2NH (excess), r.t., 5 h |
20/1 |
90 |
i-PrOH |
0 |
i-PrOH (excess), re¯ux, 18 h |
|
0 |
i-PrOH |
1.5 |
i-PrOH (excess), re¯ux, 18 h |
100/1 |
88 |
PhSH |
0 |
PhSH (5.0 eq.), benzene, r.t., 22 h |
|
0 |
PhSH |
1.5 |
PhSH (1.6 eq.), benzene, r.t., 5 min |
6.4/1 |
95 |
Me3SiN3 |
1.5 |
Me3SiN3 (3.0 eq.), benzene, re¯ux, 3 h |
14/1 |
74 |
|
|
|
|
|
r.t. ˆ Room temperature.
Reprinted with permission by Am. Chem. Soc., Ref. 21.

206 ASYMMETRIC OXIDATIONS
4.2.1.2 Regioselective Azide Opening of 2,3-Epoxy Alcohols by [Ti(OPri)2(N3)2] and Other Azidic Compounds. Similarly, an azide can be introduced into 2,3-epoxy alcohols like 23 and those shown in Table 4±5. Using Ti(OPri)2(N3)2 as a nucleophile, ring opening proceeds readily in the presence of Ti(OPri)4, yielding the C-3 ring opening product as the major product. The C-3 ring opening products can be used for preparing various a-amino acids.23
TABLE 4±5. Preparation of the Azide Compounds
Entry |
Substrate |
Conditions |
Regioselectivity C-3/C-2 |
Yield (%) |
|
|
|
|
|
1 |
|
7 ha |
5.8:1 |
95 |
2 |
|
0.08 hb |
36:1 |
88 |
3 |
|
3.5 hc |
1.4:1 |
71 |
4 |
|
0.16 hb |
27:1 |
96 |
5 |
|
10 hc |
1.7:1 |
93 |
6 |
|
0.25 hb |
20:1 |
94 |
7 |
|
12 hc |
1:100 |
47 |
8 |
|
0.75 hb |
2:1 |
96 |
9 |
|
2.75 ha |
100:1 |
100 |
10 |
|
0.08 hd |
100:1 |
76 |
a NaN3/NH4Cl, 65 C, MeOH/H2O ˆ 8:1. b Ti(OPri)2(N3)2, benzene, 70 C.
c NaN3/NH4Cl, CH3OCH2CH2OH:H2O ˆ 8:1; 124 C. d Ti(OPri)2(N3)2, ether, 25 C.
Reprinted with permission by Am. Chem. Soc., Ref. 23.
The preparation of Ti(OPri)2(N3)2, is described elsewhere.24
Besides Ti(OPri)2(N3)2, other azide compounds are also e¨ective for C-3 ring opening with 2,3-epoxy alcohols. Benedetti et al.25 have demonstrated another regioselective and stereoselective ring-opening reaction using diethyl aluminum azide as the nucleophile. High regioselectivity (C-3 ring opening over C-2 ring opening) has been observed for both cisand trans-substituted epoxides, and the C-3 attack is not a¨ected by bulky substituents at C-3.
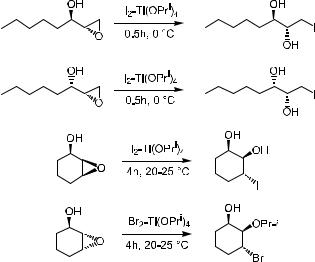
4.2 SELECTIVE OPENING OF 2,3-EPOXY ALCOHOLS |
207 |
4.2.1.3 Ring-Opening Reactions of Epoxy Alcohols with X2-Ti(OPri)4.
Treating allylic and homoallylic epoxy alcohols with an equivalent amount of halogen (Br2, I2) in the presence of a stoichiometric amount of Ti(OPri)4 provides halohydrins under mild conditions with a high degree of generality and with good regioselectivity (Scheme 4±12).26
Scheme 4±12
4.2.2Opening by Intramolecular Nucleophiles
Another approach that produces regioselective ring opening in 2,3-epoxy alcohols is to take advantage of intramolecular nucleophiles by attaching a potential N-nucleophile or O-nucleophile to the hydroxy group.27 The application of this intramolecular ring opening is exempli®ed in the synthesis of sphingosine isomers. In the enantioselective synthesis of d- and l-erythro-sphingosine,28 2,3- epoxy alcohol 26 is treated with excess CCl3CN in the presence of DBU. The resulting trichloroimidate 27 can serve as an N-nucleophile. Treating 27 with triethyl aluminum leads to a single product 28 in which the C-3 con®guration has been reversed. After acid hydrolysis and Li/NH3 reduction, l-erythro- sphingosine is obtained and can be characterized as its triacetate (30b) (Scheme 4±13).
Cerebrosides are major constituents of the membrane of brain cells. They are the simplest glycosphingolipids, serving as model substances for more complex lipids of this kind. Furthermore, they are credited with important properties as receptors for hormones and toxins.29 Schemes 4±13 and 4±14 provide a method for preparing sphingosine and its analogs that can be used for the synthesis of cerebroside compounds.

208 ASYMMETRIC OXIDATIONS
Scheme 4±13. Enantioselective synthesis of l-erythro-sphingosine.
To prepare the desired d-erythro-isomer d-30b, benzyl urethane 32 is prepared via the p-nitrophenylcarbonate 31 in a one pot reaction. Treating this N- nucleophile 32 with 5 equivalents of NaN(SiMe3)2 leads to the desired oxazolidine 33. Li/NH3 cleavage a¨ords d-erythro-sphingosine, which may also be characterized as its triacetate d-30 (Scheme 4±14).
Scheme 4±14. Enantioselective synthesis of d-erythro-sphingosine (d-30).
Intramolecular ring opening is also exempli®ed in the synthesis of …4R†-4- [(E )-2-butenyl]-4-N-dimethyl-l-threonine (MeBmt 34), an unusual b-hydroxy- a-amino acid of cyclosporine (35),30 which is a clinically used immunosuppressing agent.
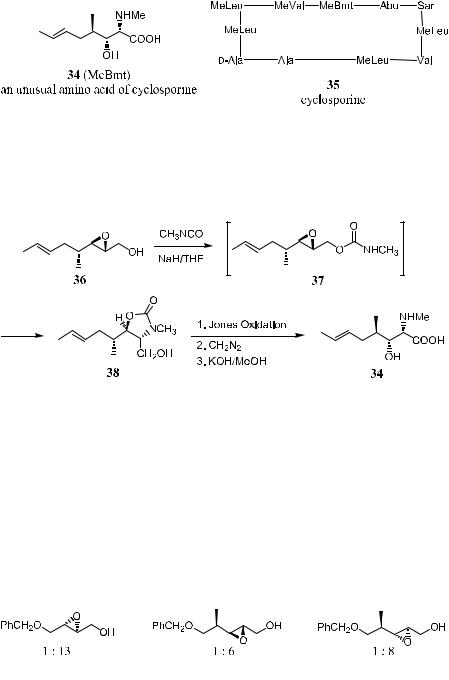
4.2 SELECTIVE OPENING OF 2,3-EPOXY ALCOHOLS |
209 |
Key steps, as shown in Scheme 4±15, involve the formation of a urethane intermediate 37 by treating epoxide 36 with methyl isocyanate in the presence of sodium hydride. Intramolecular N-nucleophilic ring opening of oxirane affords oxazolidine 38. Subsequent treatment furnishes product 34.
Scheme 4±15. Key steps in the synthesis of MeBmt.
4.2.3Opening by Metallic Hydride Reagents
Using di¨erent reagents or under various conditions, 2,3-epoxy alcohols can undergo ring-opening reactions with metallic hydrides, giving 1,3-diols or 1,2- diols. As shown in Scheme 4±16, reduction of 3-substituted 2,3-epoxy alcohols with Red-Al leads to the exclusive formation of 1,3-diols, and this can be applied in the preparation of 1,3-diol compounds.31
In contrast to Red-Al reductions, DIBAL-H or LiBH4/Ti(OPri)4 reduction of epoxides yields 1,2-diols as the major products.32 When treated with DI- BAL-H, ratios of 1,3- to 1,2-diol ranging from 1:6 to 1:13 have been observed.
An alternative method for reducing 2,3-epoxy alcohols to 1,2-diols through regioselective delivery of hydride to C-3 is realized by treating the correspond-
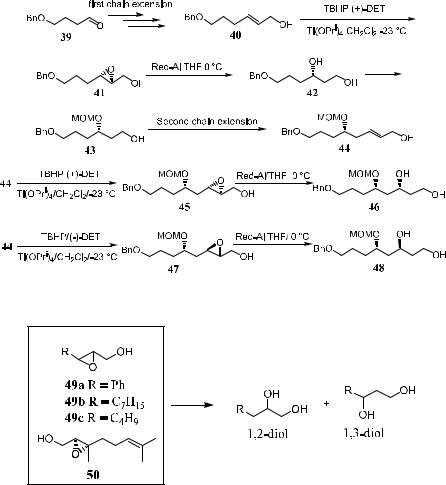
210 ASYMMETRIC OXIDATIONS
Scheme 4±16. Synthesis of 1,3-diol.
Scheme 4±17. Reduction of 2,3-epoxy alcohols by LiBH4/Ti(OPri)4.
ing epoxide compound with excess LiBH4 in the presence of Ti(OPri)4 (Scheme 4±17 and Table 4±6). This reaction carried out at low temperature gives high stereoselectivity but rather low reaction rate. Raising the reaction temperature generally results in a drop in enantioselectivity.33
A similar ring opening reported by Sajiki et al.34 involves the catalytic hydrogenolysis of terminal epoxides.
4.2.4Opening by Organometallic Compounds
When treated with organocuprates, 2,3-epoxy alcohols can be converted to substituted 1,3-diols with high regioselectivity and stereoselectivity. Thus, as