
Principles and Applications of Asymmetric Synthesis
.pdf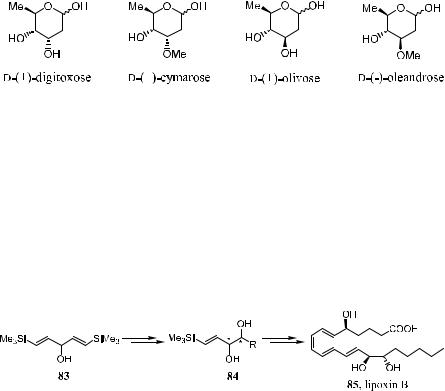
4.4 ENANTIOSELECTIVE DIHYDROXYLATION OF OLEFINS |
221 |
Figure 4±3
Schreiber's model has also proved to be a general approach to a series of oxygenated metabolites of arachidonic acid, such as lipoxin A and lipoxin B.50 The family of linear oxygenated metabolites of arachidonic acid has been implicated in immediate hypersensitivity reactions, in¯ammation, and a number of other health problems. Among these metabolites, several compounds, such as lipoxin A, lipoxin B, 5,6-diHETE, and 14,15-diHETE possess 1-substituted (E )-1-alken-3,4-diol 84 as a common substructural moiety. Therefore, the carbinol 83 is an ideal substrate for generating compound 84 by applying Sharpless epoxidation reaction.50
4.4ENANTIOSELECTIVE DIHYDROXYLATION OF OLEFINS
The history of asymmetric dihydroxylation51 dates back 1912 when Ho¨mann showed, for the ®rst time, that osmium tetroxide could be used catalytically in the presence of a secondary oxygen donor such as sodium or potassium chlorate for the cis-dihydroxylation of ole®ns.52 About 30 years later, Criegee et al.53 discovered a dramatic rate enhancement in the osmylation of alkene induced by tertiary amines, and this ®nding paved the way for asymmetric dihydroxylation of ole®ns.
The ®rst attempt to e¨ect the asymmetric cis-dihydroxylation of ole®ns with osmium tetroxide was reported in 1980 by Hentges and Sharpless.54 Taking into consideration that the rate of osmium(VI) ester formation can be accelerated by nucleophilic ligands such as pyridine, Hentges and Sharpless used l-2- (2-menthyl)-pyridine as a chiral ligand. However, the diols obtained in this way were of low enantiomeric excess (3±18% ee only). The low ee was attributed to the instability of the osmium tetroxide chiral pyridine complexes. As a result, the naturally occurring cinchona alkaloids quinine and quinidine were derived to dihydroquinine and dihydroquinidine acetate and were selected as chiral
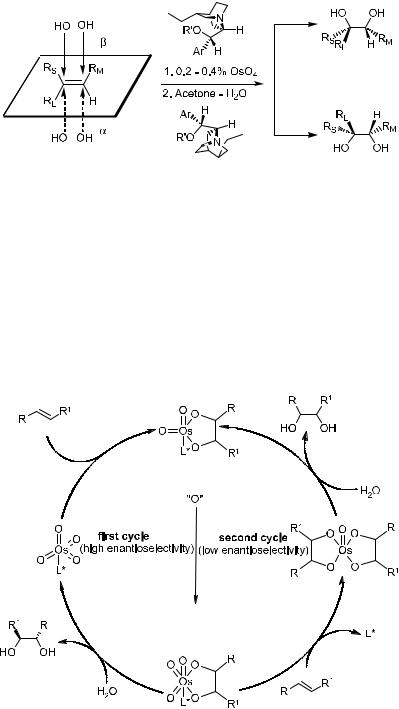
222 ASYMMETRIC OXIDATIONS
Figure 4±4. Asymmetric dihydroxylation reaction.
auxiliaries. Because the bonding of osmium tetroxide to quininuclidine nitrogen is much tighter than the bonding of chiral pyridine, the diols obtained were in reasonably high enantiomeric excess (Fig. 4±4).
The reaction mechanism is shown in Figure 4±5. This reaction is a good example of ligand-accelerated asymmetric catalysis, as the alkaloid ligands enhance the rate by one to two orders of magnitude. In the initial stages, slow addition of the ole®n is essential to obtain high ee due to a competing second catalytic cycle with low enantioselectivity. In the work of Sharpless' group, improved enantioselectivities were observed with potassium ferricyanide as the primary oxidant under alkaline (K2CO3) conditions in aqueous t-butanol. In
Figure 4±5. Mechanism of Os-mediated dihydroxylation of oli®ns.
4.4 ENANTIOSELECTIVE DIHYDROXYLATION OF OLEFINS |
223 |
this case, the second cycle is precluded because hydrolysis of the monoglycolate complex precedes reoxidation of the osmium under these conditions. In contrast to Sharpless epoxidation, which may be in¯uenced by the bulky nature of the substituents on the substrates, asymmetric dihydroxylation is successful with a broad range of substituents.
Since Sharpless' discovery of asymmetric dihydroxylation reactions of alkenes mediated by osmium tetroxide±cinchona alkaloid complexes, continuous e¨orts have been made to improve the reaction. It has been accepted that the tighter binding of the ligand with osmium tetroxide will result in better stability for the complex and improved ee in the products, and a number of chiral auxiliaries have been examined in this e¨ort. Table 4±11 (below) lists the chiral auxiliaries thus far used in asymmetric dihydroxylation of alkenes. In most cases, diamine auxiliaries provide moderate to good results (up to 90% ee).
The major breakthrough in the catalytic asymmetric dihydroxylation reactions of ole®ns was reported by Jacobsen et al.55 in 1988. Combining 9-acetoxy dihydroquinidine as the chiral auxiliary with N-methylmorphine N-oxide as the secondary oxidant in aqueous acetone produced optically active diols in excellent yields, along with e½cient catalytic turnover.
The 4-chlorobenzoate derivatives of dihydroquinidine (DHQD-CLB 91a) and dihydroquinine (DHQ-CLB 91b) have been found to yield optically active diols with high optical purity. Although several aryl-substituted alkenes yield the corresponding diols with high optical purity, reactions with alkyl-substituted ole®ns normally show lower stereoselectivity. Poor ee values are also observed for cis or cyclic ole®ns. Slow addition of the ole®ns to the reaction mixture improves the rate, as well as the ee with all diols, and all of the trans-disubstituted aromatic ole®ns can give products with ee in the range of 80±99%.56 For aliphatic cisand trans-ole®ns or terminal, tri-, and tetrasubstituted ole®ns, the ee of the products are still not high enough for the reactions to be synthetically useful.
The highest enantioselectivity in the dialkyl-substituted ole®nes has been obtained with the aryl ethers of DHQD 94a and DHQ 94b. With potassium ferricyanide as secondary oxidant, it is possible to carry out the reaction at room temperature, and slow addition of the ole®ns is not required. Under these conditions, the diols can be obtained in 85±90% yield and excellent enantioselectivity.
Although high asymmetric inductions have been obtained with various alkenes using cinchona alkaloids as chiral ligands, the exploration for better catalytic systems is still under way. In fact, even better ee values have been achieved using C2-symmetric ligands 96, 97, 99, and 101 introduced by Sharpless57±59 and colleagues and Lohray and Bhushan.60 Table 4±10 presents some results of catalytic asymmetric dihydroxylation of ole®ns. The high enantioselectivity in the dihydroxylation of various substituted ole®ns, which previously was possible only by applying stoichiometric reagents at low temperature, can now be achieved in catalytic fashion using C2-symmetric ligands 96, 97, 99, and 101 at room temperature.58 Among these chiral ligands, 101 is the superior ligand for asymmetric dihydroxylation reactions, with most ole®ns bearing aliphatic substituents or heteroatoms in the alkylic position.59
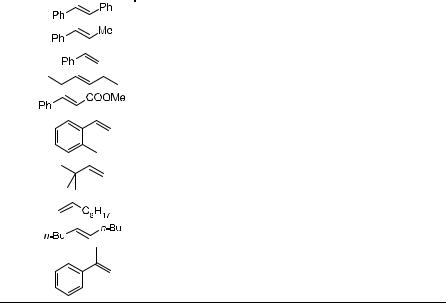
224 |
ASYMMETRIC OXIDATIONS |
|
|
|
|
TABLE 4±10. Enantiomeric Excess of Diols Obtained by Catalytic Asymmetric |
|
||||
Dihydroxylation of Alkenes61 |
|
|
|
||
|
|
|
|||
|
|
(DHQD)2-PHAL (DHQ)2-PHAL C2-DHQD C2-DHQ |
|||
Entry |
Substrate |
96a |
96b |
99 |
99 |
|
|
|
|
|
|
1 |
|
>99.5 |
>99.5 |
>98 |
98 |
2 |
|
Ð |
Ð |
>98 |
Ð |
3 |
|
97 |
97 |
92 |
85 |
4 |
|
Ð |
Ð |
93 |
Ð |
5 |
|
97 |
95 |
94 |
Ð |
6 |
|
Ð |
Ð |
76 |
Ð |
7 |
|
Ð |
Ð |
48 |
Ð |
8 |
|
84 |
80 |
45 |
Ð |
9 |
|
97 |
93 |
Ð |
Ð |
10 |
|
94 |
93 |
Ð |
Ð |
Reprinted with permission by Pergamon Press Ltd., Ref. 61.
In summary, the reaction of osmium tetroxide with alkenes is a reliable and selective transformation. Chiral diamines and cinchona alkakoid are most frequently used as chiral auxiliaries. Complexes derived from osmium tetroxide with diamines do not undergo catalytic turnover, whereas dihydroquinidine and dihydroquinine derivatives have been found to be very e¨ective catalysts for the oxidation of a variety of alkenes. OsO4 can be used catalytically in the presence of a secondary oxygen donor (e.g., H2O2, TBHP, N-methylmorpholine- N-oxide, sodium periodate, O2, sodium hypochlorite, potassium ferricyanide). Furthermore, a remarkable rate enhancement occurs with the addition of a nucleophilic ligand such as pyridine or a tertiary amine. Table 4±11 lists the preferred chiral ligands for the dihydroxylation of a variety of ole®ns.61 Table 4±12 lists the recommended ligands for each class of ole®ns.
To give a better understanding of the scope of application for epoxidation and dihydroxylation reactions in organic synthesis, the studies by several groups on these reactions are discussed in the remainder of this section.
Corey et al.66 have developed a bidentate chiral ligand 93 for asymmetric dihydroxylation of ole®ns. As shown in Table 4±13, asymmetric dihydroxylation of a series of ole®ns using 93 as a chiral catalyst and OsO4 as the oxidant gives good to excellent yield as well as good enantioselectivity in most cases.
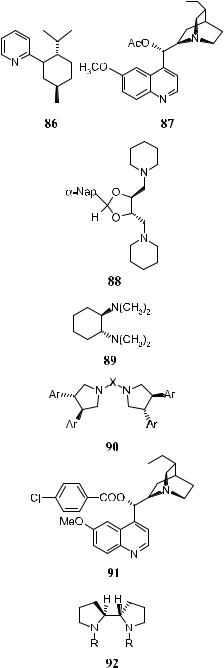
4.4 |
ENANTIOSELECTIVE DIHYDROXYLATION OF OLEFINS |
225 |
TABLE 4±11. Ligands Used in Asymmetric Dihydroxylations |
|
|
|
|
|
Ligand |
References |
|
|
|
|
54
62
63
64
55
65
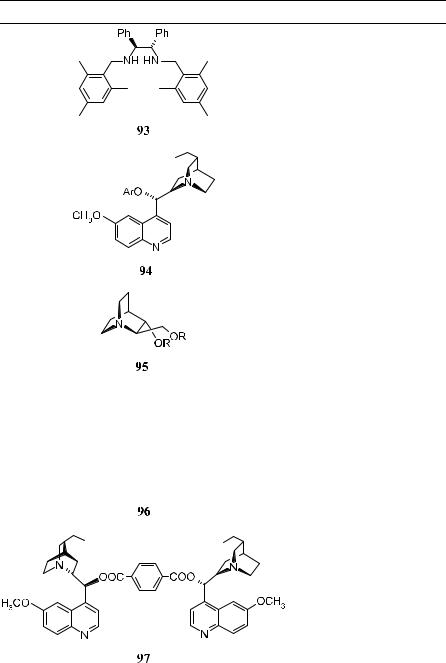
226 |
ASYMMETRIC OXIDATIONS |
TABLE 4±11 (Continued ) |
|
Ligand |
References |
|
66 |
67
68
57
60
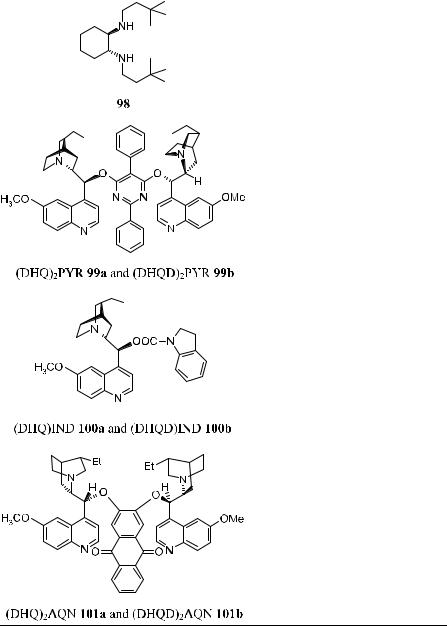
4.4 |
ENANTIOSELECTIVE DIHYDROXYLATION OF OLEFINS |
227 |
TABLE 4±11 (Continued ) |
|
|
|
|
|
Ligand |
References |
|
|
|
|
|
|
69 |
58
70
59
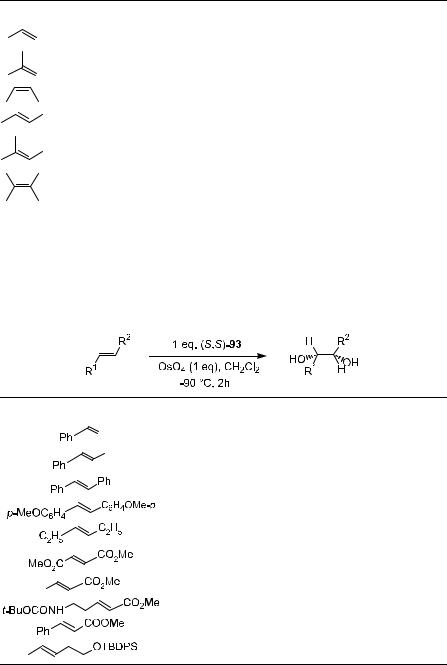
228 ASYMMETRIC OXIDATIONS
TABLE 4±12. Recommended Ligands for Each Class of Ole®ns51
Ole®n Class |
Preferred Ligand |
ee Range (%) |
|
|
|
|
PYR, PHAL |
30±97 |
|
PHAL |
70±97 |
|
IND |
20±80 |
|
PHAL |
90±99.8 |
|
PHAL |
90±99 |
|
PYR, PHAL |
20±97 |
|
|
|
ee ˆ Enantiomeric excess. |
|
|
TABLE 4±13. Enantioselective Hydroxylation of Ole®n by OsO4 N 93
Ole®n |
Yield (%) |
ee (%) |
Con®g. |
|
|
|
|
|
81 |
92 |
S |
|
95 |
93 |
S,S |
|
95 |
92 |
S,S |
|
90 |
82 |
S,S |
|
90 |
98 |
S,S |
|
75 |
92 |
2R,3R |
|
82 |
97 |
2R,3S |
|
91 |
97 |
2R,3S |
|
83 |
92 |
2R,3S |
|
87 |
95 |
2S,3S |
ee ˆ Enantiomeric excess.
Reprinted with permission by Am. Chem. Soc., Ref. 66.

4.4 ENANTIOSELECTIVE DIHYDROXYLATION OF OLEFINS |
229 |
TABLE 4±14. Enantioselective Dihydroxylation of Ole®ns Using OsO4 N 92b
Entry |
Ole®n |
Solvent |
ee (%) |
Yield (%) |
Con®g. |
|
|
|
|
|
|
1 |
(E )-stilbene |
Toluene |
100 |
96 |
S,S |
2 |
(E )-stilbene |
Acetone |
80 |
93 |
S,S |
3 |
(E )-stilbene |
CH2Cl2 |
56 |
87 |
S,S |
4 |
Ethyl (E )-3-phenylacrylate |
Toluene |
99 |
97 |
|
5 |
(E )-phenylpropene |
Toluene |
92 |
95 |
S,S |
6 |
Dimethyl fumarate |
CH2Cl2 |
98 |
79 |
R,R |
7 |
Ethyl (E )-crotonate |
CH2Cl2 |
98 |
90 |
|
8 |
(E )-2-heptene |
CH2Cl2 |
98 |
93 |
|
9 |
(E )-3-hexene |
CH2Cl2 |
96 |
82 |
S,S |
10 |
(E )-3-heptene |
CH2Cl2 |
93 |
90 |
|
11 |
(E )-1-heptene |
CH2Cl2 |
91 |
90 |
S |
12 |
Styrene |
Toluene |
88 |
90 |
S |
ee ˆ Enantiomeric excess.
Reprinted with permission by Am. Chem. Soc., Ref. 71.
Hirama and co-workers71 developed another chiral bidentate ligand 92 for OsO4-mediated dihydroxylation of trans-disubstituted and monosubstituted ole®ns. As shown in Table 4±14, asymmetric dihydroxylation of ole®ns using …S,S†-(ÿ)-92b as the chiral ligand provides excellent yield and enantioselectivity.
Chiral compounds 91a and 91b, as shown in Table 4±15, were ®rst reported by Jacobsen et al.55 for the asymmetric dihydroxylation of ole®ns. These catalysts can be used for asymmetric dihydroxlation of a variety of substrates.
Chiral catalysts (DHQD)2PHAL 96a and (DHQ)2PHAL 96b, developed by Sharpless' group, are highly e¨ective in asymmetric dihydroxylation reactions. With an oxidant and an Os source, high ee can be obtained, and the approach of hydroxyl groups can be directed to either the a- or the b-side of the prochiral face of the substrate by choosing the appropriate catalyst, 96a or 96b. Sharpless and co-workers have formulated the chiral ligand, metal, and oxidant as AD mix-a and AD mix-b. For example, a mixture of (DHQ)2PHAL, K3Fe(CN)6, K2CO3 and potassium osmate is AD mix-a. Currently, both AD mix-a and AD mix-b are commercially available.
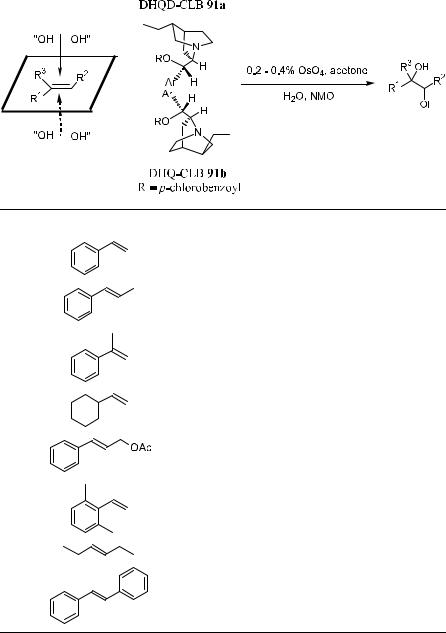
230 ASYMMETRIC OXIDATIONS
TABLE 4±15. Asymmetric Dihydroxylation of Ole®ns with OsO4 Induced by 91a or 91b
Entry |
Ole®n |
Ligand |
ee (%) |
Con®g. |
Sign of [a]D |
Time (h) |
1 |
|
91a |
62 |
R |
(ÿ) |
3 |
|
|
91a |
60 |
R |
(ÿ) |
7 |
|
|
91b |
53.6 |
S |
(‡) |
7 |
2 |
|
91a |
65 |
R,R |
(ÿ) |
5 |
|
|
91b |
55.4 |
S,S |
(‡) |
12 |
3 |
|
91a |
33 |
R |
(ÿ) |
1.5 |
4 |
|
91a |
46 |
R |
(‡) |
1 |
5 |
|
91a |
76 |
R |
(‡) |
7 |
6 |
|
91a |
65 |
|
(ÿ) |
3 |
7 |
|
91a |
20 |
R,R |
(‡) |
17 |
8 |
|
91a |
88 |
R,R |
(‡) |
7 |
|
|
91a |
85 |
R,R |
(‡) |
15 |
|
|
91b |
78.5 |
S,S |
(ÿ) |
17 |
ee ˆ Enantiomeric excess.
Reprinted with permission by Am. Chem. Soc., Ref. 55.