
Wasserscheid P., Welton T. - Ionic Liquids in Synthesis (2002)(en)
.pdf
|
|
Ionic Liquids in Synthesis. Edited by Peter Wasserscheid, Thomas Welton |
|
|
Copyright © 2002 Wiley-VCH Verlag GmbH & Co. KGaA |
336 |
1 Kolumnentitel |
ISBNs: 3-527-30515-7 (Hardback); 3-527-60070-1 (Electronic) |
|
|
|
8
Biocatalytic Reactions in Ionic Liquids
Udo Kragl, Marrit Eckstein, and Nicole Kaftzik
8.1
Introduction
Biocatalytic reactions and production processes have been established as useful tools for several decades. The Reichstein process for the oxidation of D-sorbitol to L- sorbose by the use of whole microorganisms, which is still in use, was introduced as early as 1934 [1]. Several years ago, BASF introduced a lipase-catalyzed process for the kinetic resolution of chiral amines [2]. During the history of biocatalysis, alternative reaction conditions have been investigated with the goals of overcoming such problems as substrate solubility, selectivity, yield, or catalyst stability. Some progress has been made through the use of organic solvents [3, 4], the addition of high salt concentrations [5], and the use of microemulsions [6] or supercritical fluids [7]. Recently the methods of gene technology – site-directed mutagenesis and directed evolution – have added new and powerful tools for the development of better biocatalysts [8, 9]. It was thus unsurprising that researchers in the field of biocatalysis have begun to focus on ionic liquids as novel solvents in order to find new solutions to known problems.
In this chapter, we try to summarize the work so far reported in this field. We first give a short introduction into the different forms of biocatalytic reactions, highlighting some special properties of biocatalysts.
8.2
Biocatalytic Reactions and their Special Needs
Biotechnological processes may be divided into fermentation processes and biotransformations. In a fermentation process, products are formed from components in the fermentation broth, as primary or secondary metabolites, by microorganisms or higher cells. Product examples are amino acids, vitamins, or antibiotics such as penicillin or cephalosporin. In these cases, co-solvents are sometimes used for in situ product extraction.

8.2 Biocatalytic Reactions and their Special Needs 337
The term “biotransformation” or “biocatalysis” is used for processes in which a starting material (precursor) is converted into the desired product in just one step. This can be done by use either of whole cells or of (partially) purified enzymes. Product examples range from bulk chemicals (such as acrylamide) to fine chemicals and chiral synthons (chiral amines or alcohols, for example). There are several books and reviews dealing with the use of biotransformations either at laboratory or at industrial scales [1, 10–13].
Biocatalysts in nature tend to be optimized to perform best in aqueous environments, at neutral pH, temperatures below 40 °C, and at low osmotic pressure. These conditions are sometimes in conflict with the need of the chemist or process engineer to optimize a reaction with respect to space-time yield or high product concentration in order to facilitate downstream processing. Furthermore, enzymes and whole cells are often inhibited by products or substrates. This might be overcome by the use of continuously operated stirred tank reactors, fed-batch reactors, or reactors with in situ product removal [14, 15]. The addition of organic solvents to increase the solubility of substrates and/or products is a common practice [16].
Generally, there are three ways to use organic solvents or ionic liquids in a biocatalytic process:
1.as a pure solvent,
2.as a co-solvent in aqueous systems, or
3.in a biphasic system.
When either the organic solvent or the ionic liquid is used as pure solvent, proper control over the water content, or rather the water activity, is of crucial importance, as a minimum amount is necessary to maintain the enzyme’s activity. For ionic liquids, a reaction can be operated at constant water activity by use of the same methods as established for organic solvents [17]. [BMIM][PF6] or [BMIM][(CF3SO2)2N], for example, may be used as pure solvents and in biphasic systems. Water-miscible ionic liquids, such as [BMIM][BF4] or [MMIM][MeSO4], can be used in the second case.
It should be noted that, despite the success of the application of conventional organic solvents, there is no general rule as to which solvent is “enzyme friendly”. To a certain extent, the log P concept, based on the distribution coefficient between water and octanol, can be used as guideline [18]. In general, solvents with a log P value greater than 3, such as xylene (3.1) or hexane (3.9), are less deactivating than those with a low log P value, such as ethanol (–0.24). Certainly, the hydrophilicity of the co-solvent is important, as it allows interaction with and breaking of hydrogen bonds that stabilize the tertiary structure of the protein. Such interactions are very likely to occur with ionic liquids as well. Surprisingly, enzymes and even whole cells are active in various ionic liquids, as shown in Section 8.3. So far, ionic liquids have not been treated according to the log P concept. However, the polarities of ionic liquids have been investigated by different groups [19–22]. The polarities of different ionic liquids such as [BMIM][PF6] or [EMIM][(CF3SO2)2N] are similar to those of polar solvents such as ethanol or N-methylformamide. On Reichardt’s normalized polarity scale, ranging from 0 for tetramethylsilane to 1 for water, ionic liquids have

338 Udo Kragl, Marrit Eckstein, Nicole Kaftzik
polarities around 0.6. Toluene (0.1) and MTBE (0.35) are less polar [22, 21] (for more details on the polarity of ionic liquids see Section 3.5). Both of these solvents are commonly used as water-immiscible solvents in enzyme catalysis. When used with whole cells, organic solvents often damage the cell membrane. So far, only little is known about the toxicity of ionic liquids, although LD50 values of 1400 mg kg–1 in female Wistar rats have recently been reported for 3-hexoyloxymethyl-1-methylimi- dazolium tetrafluoroborate [23]. From this the authors concluded that tetrafluoroborates could be used safely.
When starting our first experiments with available ionic liquids, in screening programs to identify suitable systems, we encountered several difficulties such as pH shifts or precipitation. More generally, the following aspects should be taken into account when ionic liquids are used with biocatalysts:
●In some cases, impurities in the ionic liquids resulted in dramatic pH shifts, causing enzyme inactivation. This could sometimes be overcome simply by titration or higher buffer concentrations. In other cases, purification of the ionic liquid or an improved synthesis might be necessary.
●Enzymatic reactions are often performed in aqueous buffer solution; addition of increasing amounts of ionic liquids sometimes caused precipitates of unknown composition.
●To maintain enzymatic activity a minimal amount of water has to be present, best described by the water activity. However, water present in the reaction system may cause hydrolysis of some ionic liquids.
●Some enzymes require metal ions – such as cobalt, manganese or zinc – for their activity; if these are removed by the ionic liquid by complexation, enzyme inactivation may occur.
●Impurities or the ions of the liquid themselves may act as reversible or irreversible enzyme inhibitors.
●For kinetic investigations and for activity measurements, either photometric assays or – because of the higher complexity of the reactants converted by biocatalysts – HPLC methods can often be used. Here the ionic liquid itself or impurities may interfere with the analytical method.
●Unlike in the case of conventional organic solvents, most research groups prepare the ionic liquids themselves. This may be the reason why different results are sometimes obtained with the same ionic liquids. Park and Kazlauskas performed a washing procedure with aqueous sodium carbonate and found improved reaction rates, but this might also be related to a more precisely defined water content/water activity in the reaction system [22].
As with organic solvents, proteins are not soluble in most of the ionic liquids when they are used as pure solvent. As a result, the enzyme is either applied in immobilized form, coupled to a support, or as a suspension in its native form. For production processes, the majority of enzymes are used as immobilized catalysts in order to facilitate handling and to improve their operational stability [24–26]. As support, either inorganic materials such as porous glass or different organic polymers are used [27]. These heterogeneous catalyst particles are subject to internal and external

8.3 Examples of Biocatalytic Reactions in Ionic Liquids 339
mass transport limitations, which are strongly influenced by the viscosity of the reaction medium. For [BMIM][(CF3SO2)2N], a dynamic viscosity of 52 mPa s at 20 °C has been reported [19]. For comparison, MTBE has a viscosity of only 0.34 mPa s. The viscosity can be reduced to a large extent by increasing the temperature or by addition of small amounts of an organic solvent [28] (for more information on viscosity of ionic liquids, see Section 3.2). This important aspect of the use of ionic liquids in biocatalysis warrants further study.
8.3
Examples of Biocatalytic Reactions in Ionic Liquids
Thanks to their special properties and potential advantages, ionic liquids may be interesting solvents for biocatalytic reactions to solve some of the problems discussed above. After initial trials more than 15 years ago, in which ethylammonium nitrate was used in salt/water mixtures [29], results from the use of ionic liquids as pure solvent, as co-solvent, or for biphasic systems have recently been reported. The reaction systems are summarized in Tables 8.3-1 and 8.3-2, below. Table 8.3-1 compiles all biocatalytic systems except lipases, which are shown separately in 8.3-2. Some of the entries are discussed in more detail below.
8.3.1
Whole-cell Systems and Enzymes other than Lipases in Ionic Liquids
In 1984, Magnuson et al. (Entry 1) investigated the influence of ethylammonium/water mixtures on enzyme activity and stability [29]. At low [H3NEt][NO3] concentrations, an increased activity of alkaline phosphatase was found. The same ionic liquid was used by Flowers and co-workers, who found improved protein refolding after denaturation (Entry 2) [30].
So far only two groups have reported details of the use of ionic liquids with wholecell systems (Entries 3 and 4) [31, 32]. In both cases, [BMIM][PF6] was used in a twophase system as substrate reservoir and/or for in situ removal of the product formed, thereby increasing the catalyst productivity. Scheme 8.3-1 shows the reduction of ketones with bakers’ yeast in the [BMIM][PF6]/water system.
The recovery of n-butanol from a fermentation broth in a similar way has been investigated by in situ extraction with [BMIM][PF6] (Entry 5) [33].
In the first publication describing the preparative use of an enzymatic reaction in ionic liquids, Erbeldinger et al. reported the use of the protease thermolysin for the synthesis of the dipeptide Z-aspartame (Entry 6) [34]. The reaction rates were comparable to those found in conventional organic solvents such as ethyl acetate. Additionally, the enzyme stability was increased in the ionic liquid. The ionic liquid was recycled several times after the removal of non-converted substrates by extraction with water and product precipitation. Recycling of the enzyme has not been reported. It should be noted, however, that according to the log P concept described in the previous section, ethyl acetate – with a value of 0.68 – may interfere with the pro-

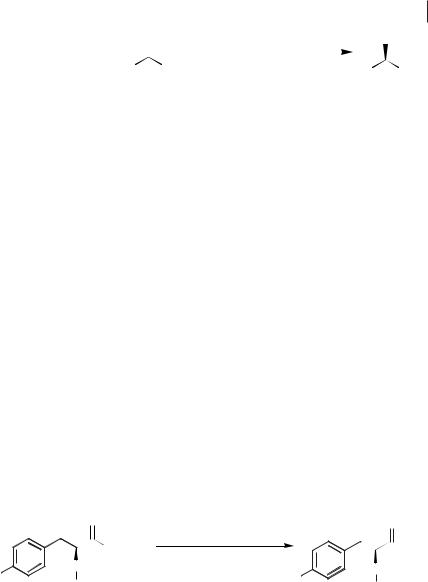
8.3 Examples of Biocatalytic Reactions in Ionic Liquids 341
Scheme 8.3-1 |
|
O |
|
baker’s yeast; MeOH |
|
|
OH |
||
|
|
|
|
|
|
|
|||
|
|
|
|
|
|
|
|
|
|
|
|
|
|
Me [BMIM][PF6]:H2O (10:1) |
|
|
|
||
|
R |
R |
|
Me |
|||||
|
|
|
|
|
33°C; 72h |
|
|
|
|
|
R = -C4H9 (yield 22%, eeS 95%) |
|
[32] |
||||||
|
R = -CH2-COOEt (yield 75%, eeS 84%) |
|
|
|
tein in an undesired way. The commercial production process for aspartame uses the soluble enzyme in an aqueous system [1].
The protease α-chymotrypsin has been used for transesterification reactions by two groups (Entries 7 and 8) [35, 36]. N-Acetyl-l-phenylalanine ethyl ester and N- acetyl-l-tyrosine ethyl ester were transformed into the corresponding propyl esters (Scheme 8.3-2).
Laszlo and Compton used [OMIM][PF6] and [BMIM][PF6] and compared the results with those obtained with other organic solvents such as acetonitrile or hexane (Entry 7) [35]. They also investigated the influence of the water content on enzyme activity, as well as on the ratio of transesterification and hydrolysis. They found that, as with polar organic solvents, a certain amount of water was necessary to maintain enzymatic activity. For both ionic liquids and organic solvents, the rates were of the same order of magnitude. No data concerning the recycling of the enzyme or its stability were given.
Iborra and co-workers (Entry 8) examined the transesterification of N-acetyl-L- tyrosine ethyl ester in different ionic liquids and compared their stabilizing effect relative to that found with 1-propanol as solvent [36]. Despite the fact that the enzyme activity in the ionic liquids tested reached only 10 to 50 % of the value in 1- propanol, the increased stability resulted in higher final product concentrations. Fixed water contents were used in both studies.
O |
O |
|
|
α-Chymotrypsin |
|
OEt |
OPr |
|
NH |
1-propanol |
|
NH |
||
R |
||
R |
||
Ac |
Ac |
|
|
[35]: ionic liquid (up to 1.0% v/v H2O); 40°C
|
R= H |
|
N-acetyl-L-phenylalanine |
|
ethyl ester |
|
[36]: ionic liquid (2% v/v H2O); 50°C |
|
R= OH |
|
N-acetyl-L-tyrosine |
Scheme 8.3-2 |
ethyl ester |

342 Udo Kragl, Marrit Eckstein, Nicole Kaftzik
Husum et al. found that the hydrolytic activities of β-galactosidase from E. coli and the protease subtilisin in a 50 % aqueous solution of the water-miscible ionic liquid [BMIM][BF4] were comparable to those in 50 % aqueous solutions of ethanol or acetonitrile (Entry 9) [37].
We have studied transfer galactosylation with β-galactosidase from Bacillus circulans for the synthesis of N-acetyl-lactosamine, starting from lactose and N-acetyl- glucosamine (Entry 10) [38]. When the reaction is performed in an aqueous system, the problem of this approach is the secondary hydrolysis of the product by the same enzyme. As a consequence, yields are less than 30 %, and it is important to separate enzyme and product when the maximum yield is obtained. Through the addition of 25 % v/v of [MMIM][MeSO4] as a water-miscible co-solvent, the secondary hydrolysis of the product formed is effectively suppressed, resulting in a doubling of the yield to almost 60 %! Kinetic studies demonstrated that the enzyme activity was not influenced by the presence of the ionic liquid. The enzyme is stable under the conditions employed, allowing its repeated use after filtration with a commercially available ultrafiltration membrane. Further studies to explain the observed effect through analysis of the water activity are underway.
Entries 7, 8, and 10 describe so-called kinetically controlled syntheses starting from activated substrates such as ethyl esters or lactose. In two reaction systems it was possible to demonstrate that ionic liquids can also be useful in a thermodynamically controlled synthesis starting with the single components (Entry 11) [39]. In both cases, as with the results presented in entry 6, the ionic liquids were used with addition of less than 1 % water, necessary to maintain the enzyme activity. The yields observed were similar or better than those obtained with conventional organic solvents.
In order to broaden the field of biocatalysis in ionic liquids, other enzyme classes have also been screened. Of special interest are oxidoreductases for the enantioselective reduction of prochiral ketones [40]. Formate dehydrogenase from Candida boidinii was found to be stable and active in mixtures of [MMIM][MeSO4] with buffer (Entry 12) [41]. So far, however, we have not been able to find an alcohol dehydrogenase that is active in the presence of ionic liquids in order to make use of another advantage of ionic liquids: that they increase the solubility of hydrophobic compounds in aqueous systems. On addition of 40 % v/v of [MMIM][MeSO4] to water, for example, the solubility of acetophenone is increased from 20 mmol L–1 to 200 mmol L–1.
8.3.2
Lipases in Ionic Liquids
The majority of enzymes reported so far to be active in ionic liquids belong to the class of lipases, the “work horses” of biocatalysis [11]. Designed in nature to work at aqueous/organic interfaces for the cleavage of fats and oils, making the cleavage products accessible as nutrients, lipases in general tolerate and are active in pure organic solvents. This concept has been pioneered by Klibanov and co-work- ers [16, 42].
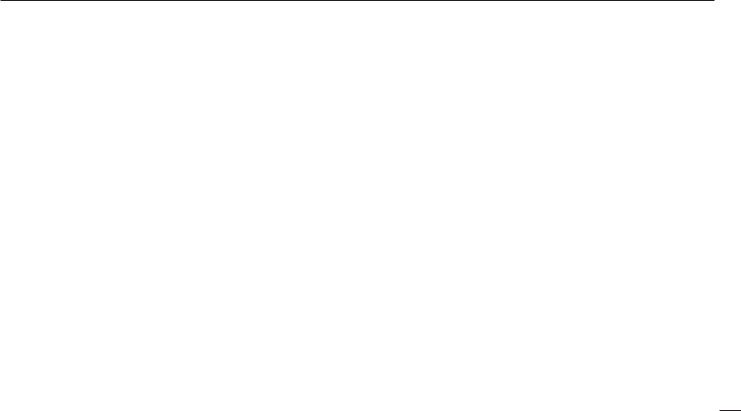
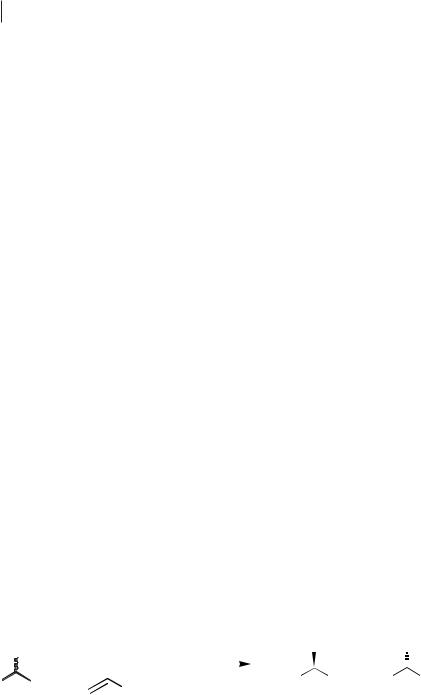

8.4 Conclusions and Outlook 345
OH |
|
Lipase |
OAc |
|
|
|
|
|
OAc |
|
|
|
|||
|
|
|
|
|
|
|
|
|
|
|
|
|
|
|
|
O |
|
Candida antarctica |
|
O |
|
|
|
|
+ |
|
O |
|
|
|
|
|
|
|
|
|
|
|
|
|
|
||||||
|
|
|
|
|
|
|
|
|
|
|
|||||
HO |
|
|
HO |
|
|
|
|
HO |
|
|
|
||||
|
|
|
|
|
|
|
|
|
|||||||
HO |
OH |
OAc , ionic liquid |
HO |
|
|
|
|
|
AcO |
|
|
|
|
||
OH |
|
55°C; 36h |
|
OH |
|
|
|
OH |
|
OH |
|
|
|
OH |
|
|
|
|
|
|
|
|
|
|
|
|
|
||||
β-D-glucose |
|
|
6-O-acetyl D-glucose |
|
3,6-O-diacetyl D-glucose |
||||||||||
|
|
|
(mixture of anomers) |
|
(mixture of anomers) |
example: 1-Methoxyethyl-3-methylimidazolium ([MOEMIM]) [BF4] dissolves ~5mg/ml glucose at 55°C yield 99%; selectivity: 93% 6-O-acetyl D-glucose [22]
Scheme 8.3-4
method of water activity equilibration over saturated salt solutions [49] and were able to demonstrate that, in contrast to MTBE, which is commonly used for this type of reaction, the enantioselectivity of the lipase was less influenced either by the water content or by the temperature when the reaction was performed in [BMIM][(CF3SO2)2N].
8.4
Conclusions and Outlook
The results reported so far clearly demonstrate the potential of ionic liquids as solvents for biotransformations. The possible variations for tailor-made solvents may have an impact similar to that of the pioneering work of Klibanov in the use of enzymes in pure organic solvents [42]. Further studies are necessary to identify the reasons for the effects observed, such as better stability, selectivity, or suppression of side reactions. Because of their ionic nature, ionic liquids might interact with charged groups in the enzyme, either in the active site or at its periphery, causing changes in the enzyme’s structure. To use ionic liquids in biocatalytic reactions in some cases requires special properties or purities, in order – for example – to avoid changes in the pH of the reaction medium.
When ionic liquids are used as replacements for organic solvents in processes with nonvolatile products, downstream processing may become complicated. This may apply to many biotransformations in which the better selectivity of the biocatalyst is used to transform more complex molecules. In such cases, product isolation can be achieved by, for example, extraction with supercritical CO2 [50]. Recently, membrane processes such as pervaporation and nanofiltration have been used. The use of pervaporation for less volatile compounds such as phenylethanol has been reported by Crespo and co-workers [51]. We have developed a separation process based on nanofiltration [52, 53] which is especially well suited for isolation of nonvolatile compounds such as carbohydrates or charged compounds. It may also be used for easy recovery and/or purification of ionic liquids.
There is still a long way to go before ionic liquids can become commonly used in biocatalysis. This will require: