
Multiple Bonds Between Metal Atoms / 12-Rhodium Compounds
.pdf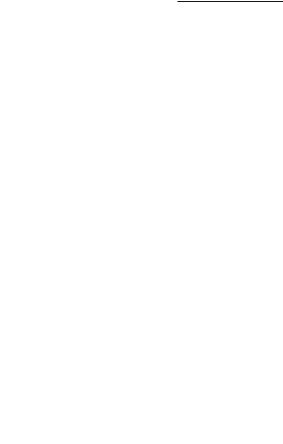
Rhodium Compounds 535
Chifotides and Dunbar
Irradiation of the cation [Rh2(bridge)4]2+, dissolved in acidic aqueous solutions (12 M HCl) at 550 nm, produces H2 and [Rh2(bridge)4Cl2]2+.646 The photochemical release of H2 from water has been the subject of detailed mechanistic studies due to the interest stemming from the point of view of energy storage.12,651-655 It has been proposed that the system initially involves reaction of [Rh2(bridge)4]2+ with H+ to form H2 and the tetranuclear cluster [Rh2(bridge)4Cl]24+ followed by a photochemical reaction that converts the latter to [Rh2(bridge)4Cl2]2+.12 The structure of the tetranuclear cluster H3[Rh4(bridge)8Cl][CoCl4]4·6H2O, which is a derivative of the chloride deficient [Rh4(bridge)8Cl]5+ cation, has been crystallographically determined;653 this complex is discussed with the rhodium blues in Section 12.5.2.
A number of dirhodium complexes of the type 12.47, that are bridged by two trans Ph2PCH2PPh2 (dppm) or Ph2AsCH2AsPh2 (dpam) ligands and contain monodentate isocyanide groups, have been reported.511,512,656 Electrochemical studies have established that oxidation of [Rh2(CNR)4(dppm)2]2+ is facilitated in the presence of various nitrogenous bases B, due to the increased stability of the [Rh2(CNR)4(dppm)2B2]4+ cation.657,658 Dirhodium compounds formulated as [Rh2(TMB)2(dppm)2X2]2+ (X = Cl, Br or I) and [Rh2(dimen)2(dppm)2Cl2]2+, which are supported by two trans dppm groups and the isocyanide ligands 12.44 and 12.45, respectively, have also been reported.659,660
|
12.47 |
A |
series of paramagnetic complexes of the type [Rh2(µ-Z)(CNBut)2(µ-LL')2](PF6)2 |
(Z = |
pyrazolate (12.9) or substituted pyrazolate ligand; LL' = Ph2PCH2PPh2 (dppm), |
Ph2PCH2AsPh2 (dpapm) or Ph2AsCH2AsPh2 (dpam)) with a Rh23+ core and a mixed set of ligands have been prepared by controlled-potential electrolysis of the Rh22+ compounds [Rh2(µ-Z)(CNBut)2(µ-LL')2]PF6.661 The two-electron oxidation of Rh22+ compounds to the Rh24+ analogs [Rh2(µ-Z)(CNBut)2(µ-dppm)2X2]PF6 (X = Cl−, Br− I−, SCN−, NO3− or CH3CO2−) has been electrochemically performed by treatment with halogens, HNO3, CH3CO2H or disproportionation of the parent Rh23+ compound in the presence of anionic ligands.662 An important structural feature of trans-[Rh2(µ-pz)(CNBut)2(µ-dppm)2Cl2]PF6662 (Fig. 12.30) is the orientation of the methylene moieties of the transoid bridging dppm ligands, which are folded away from the pyrazolate ligand, contrary to other trans dppm-bridged A-frame compounds. The analogous diiodide complex trans-[Rh2(µ-pz)(CNBut)2(dppm)2I2]BF4 has been reported as well.663
It is notable that the existence of stable dinuclear M–M bonded complexes with isocyanide groups in eq positions, even in the absence of bridging ligands, is unique to Rh24+ compounds.265 Reaction of Cr24+, Mo24+, W24+ or Re26+ compounds, not supported by bridging groups, with isocyanide ligands results in disruption of the M–M bond and formation of mononuclear products.664-666
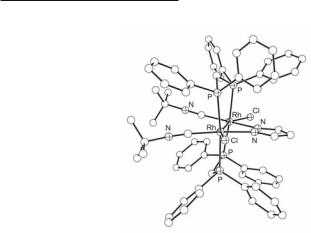
536Multiple Bonds Between Metal Atoms Chapter 12
Fig. 12.30. The cation in trans-[Rh2(µ-pz)(CNBut)2(µ-dppm)2Cl2]PF6.
12.5.2 Rhodium blues
Unlike platinum blues, which have been extensively studied (Section 14.4.7), the chemistry of the related rhodium blues is still at its nascence. Essential features of this class of compounds called ‘blues’, regardless of their color, are that they are tetranuclear (or oligonuclear) chains with at least one unsupported Rh–Rh bond, wherein the metal atoms possess non-integral oxidation numbers.667 Special requirements apply to the ligands involved in the assembly of the chain. The rhodium blues that have been the subject of single crystal X-ray studies are listed in Table 12.8.
Mixed valence molecular wire [Rh(NCCH3)4(BF4)1.5] .
A remarkable example of a rhodium blue compound is that of the unprecedented mixed valence molecular wire [Rh(NCCH3)4(BF4)1.5] (Fig. 12.31).598,605 Electrochemical reduction of [Rh2(NCCH3)10](BF4)4 at low currents produces single crystals of the 1-D chain polymeric product [Rh(NCCH3)4(BF4)1.5] at the Pt electrode over a period of three weeks. This is the first example of an infinite metal-containing chain synthesized from a dimetal precursor.668 Relevant features of [Rh(NCCH3)4(BF4)1.5] are the two different Rh–Rh interactions of 2.844(1) and 2.928(1) Å present in the chain.598,605 Both distances are significantly longer than the Rh–Rh single bond in [Rh2(NCCH3)10](BF4)4 (2.624(1) Å), but considerably shorter than the typical Rh(I)-Rh(I) contacts of c. 3.16 Å found in [Rh(CO)2(NCCH3)2]+ which forms 1-D stacks in the solid state.598 These results offer credible evidence for description of the material as being composed of Rh atoms in an average oxidation state +1.5. A view of the crystal packing is shown in Fig. 12.32. There are two different sets of torsional angles for the eq CH3CN ligands coordinated to the square planar rhodium ions, namely 44.8° between the Rh atoms with the shorter contacts and 15.3° between the Rh atoms at longer separations. A slight bending of the CH3CN ligands away from each other in the ‘dimer’ with the smaller torsion angle suggests that a minor steric effect is operative at ρ = 15.5°, which is alleviated when ρ = 44.8°.598,605
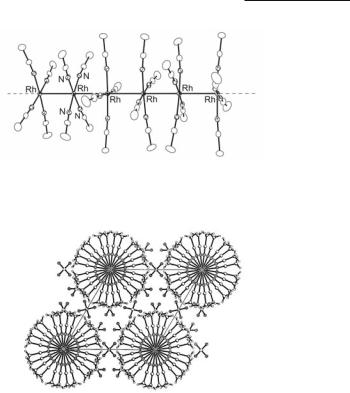
Rhodium Compounds 537
Chifotides and Dunbar
Fig. 12.31. Structure of the segment [Rh6(NCCH3)24]9+ in the molecular wire [Rh(NCCH3)4(BF4)1.5] .
Fig. 12.32. Packing of [Rh(NCCH3)4(BF4)1.5] along the c axis.
H3[Rh4(bridge)8Cl][CoCl4]4·6H2O
This rhodium blue compound contains the oxygenand light-sensitive mixed-valence cation [Rh4(bridge)8Cl]5+ 12 (bridge: 1,3-di-isocyanopropane, 12.44), which was crystallized as the tetranuclear green complex H3[Rh4(bridge)8Cl][CoCl4]4·6H2O.653 This salt is obtained by addition of CoCl2·6H2O to a solution of the photoactive complex Rh2(bridge)4(BF4)2 in 12 M HCl. The cation [Rh4(bridge)8Cl]5+ is implicated in the visible light production of H2 from aqueous acid solutions (see Section 12.5.1). It consists of two [Rh2(bridge)4]3+ units linked by a Rh–Rh bond. The Rh(II)···Rh(II) separation of 2.775(4) Å between the dinuclear units is shorter than the Rh(I)···Rh(II) separations of 2.932(4) and 2.923(3) Å within the Rh23+ units, giving rise to a Rh(I)Rh(II)Rh(II)Rh(I) chain with an average oxidation state of +1.5 for each of the four Rh atoms in the tetranuclear unit.
Reduction of the tetranuclear Rh46+ unit produces higher nuclearity oligomers including Rh68+, Rh810+ and Rh1216+ cores with nonintegral average oxidation states of the metal atoms.12,669 Despite the isolation of only few oligomers in the solid state,669 the tetranuclear clusters and their higher homologs have been characterized by various spectroscopic669,670 and electrochemical techniques.671
{[Rh(µ-pz)(CNBut)2]4}(PF6)2
Mixing equimolar amounts of the recently reported485,672 yellow compounds [Rh(I)- (µ-pz)(CNBut)2]2 and [Rh(II)(µ-pz)(CNBut)2]2(PF6)2 affords blue, EPR-silent solutions from which crystals of the tetranuclear rhodium blue complex {[Rh(µ-pz)(CNBut)2]4}(PF6)2 (Fig. 12.33) are isolated.673 The success of this synthetic approach, which involves condensation
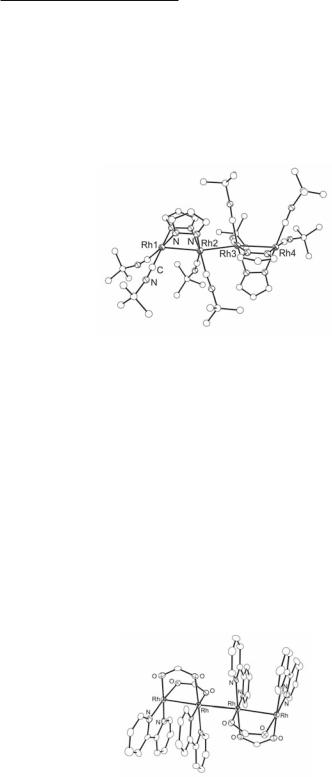
538Multiple Bonds Between Metal Atoms Chapter 12
of dinuclear complexes with Rh atoms in different oxidation states, arises from the nucleophilic character of the Rh22+ entity induced by the very basic CNBut ligands, 674 as well as by the presence of a vacant coordination site or a labile ligand trans to the Rh–Rh bond in the Rh24+ unit.667 The unsupported Rh–Rh bond of 2.713(4) Å between the {[Rh(µ-pz)(CNBut)2]4} moieties is slightly shorter by 0.01 Å than those of 2.721(4) and 2.723(4) Å encountered within the dimers and, the average oxidation state of the Rh centers is +1.5. The two dinuclear moieties are in a staggered conformation, a situation which allows the two metals to form the Rh(2)–Rh(3) bond; the three metal bonds in the crystal structure are nearly linear.
Fig. 12.33. The cation in {[Rh(pz)(CNBut)2]4}(PF6)2.
[Rh3(CNCH2Ph)12I2]Br3
Another compound with mixed valence Rh atoms and ligands of the isocyanide family is [Rh3(CNCH2Ph)12I2]Br3.675 Each Rh atom is in a pseudooctahedral environment with four isocyanide ligands located at the corners of the square. The I–Rh–Rh–Rh–I unit is nearly linear with a Rh–Rh distance of 2.785(2) Å, which is the same as that in the related compound [Rh2(CN-p-tol)8I2](PF6)2 (2.785(2) Å).601
[Rh4(O2CH)4(bpy)4](PF6)2
The tetranuclear Rh(I)–Rh(II) complex [Rh4(O2CH)4(bpy)4](PF6)2 (Fig. 12.34) is prepared under an Ar atmosphere by heating Na4[Rh2(CO)3]4 with bpy in a 10% aqueous solution of HCOOH. The molecule consists of two [Rh2(O2CH)2(bpy)2]+ entities with a Rh–Rh separation between the dinuclear units of 2.780(1) Å.235 The two rhodium atoms are bridged by two formato ligands with a Rh–Rh distance of 2.668(1) Å, which is among the shortest distances known for a Rh–Rh bond order of 0.5. The dinuclear units are linked in a transoid arrangement and the eq planes of the two rhodium atoms are partially staggered.235
Fig. 12.34. The cation in [Rh4(O2CH)4(bpy)4](PF6)2.

The molecular wire {[Rh2(O2CCH3)2(phen)2]PF6} 677 (Fig. 12.35) consists of dinuclear units linked in an infinite chain with unbridged Rh···Rh separations of 2.739(1) and 2.832(1) Å. The Rh–Rh distance of 2.652(1) Å within the [Rh2(O2CCH3)2(phen)2]+ units is the shortest known for a bond order of 0.5 and similar to the Rh(II)–Rh(II) distance of 2.624(1) Å for [Rh2(NCCH3)10]4+.597,598 The chelating phen ligands occupy transoid coordination sites of adjacent dirhodium units.
Fig. 12.35. A segment of the cationic chain in {[Rh2(O2CCH3)2(phen)2]PF6} .
{[Rh2(O2CCH3)2(bpy)2]BF4} . This infinite chain is formed by association of dinuclear {[Rh2(O2CCH3)2(bpy)2]+ ions,678 in which the rhodium atoms are bridged by two nearly eclipsed acetate ligands with a Rh–Rh distance of 2.666(2) Å. Unsupported Rh–Rh interactions between dinuclear entities display a distance of 2.833(2) Å. Similarly to {[Rh2(O2CCH3)2(phen)2]PF6} , the chelating bpy ligands on adjacent dirhodium units are in a transoid arrangement along the chain.
{[Rh4(O2CH)4(bpy)4]BF4} . This infinite chain is formed by polymerization of tetranuclear linear [Rh4(O2CH)4(bpy)2]+ units. It is the first metallic wire with the rhodium atoms in the +1.25 oxidation state.358 The Rh–Rh distances of 2.678(3) and 2.733(4) Å are encountered in the tetranuclear fragment [Rh4(O2CH)4(bpy)2]+, the shorter distance corresponding to the entity supported by the formato bridge. The molecular structure of {[Rh4(O2CH)4(bpy)4]BF4} is similar to those of {[Rh2(O2CCH3)2(bpy)2]BF4} and {[Rh2(O2CCH3)2(phen)2]PF6} .
{Rh2(O2CCF3)4[Rh2(µ-O2CCF3)2(CO)4]2} 50,310
Although this remarkable compound is not a rhodium blue, it is included in this section because it consists of arrays of six rhodium atoms linked into infinite chains (Fig. 12.8); there is some degree of electron delocalization along the chain.50 The Rh–Rh bond distance in Rh2(O2CCF3)4 is 2.412(1) Å, which is within the range of typical distances (2.35−2.45 Å) for tetracarboxylate

540Multiple Bonds Between Metal Atoms Chapter 12
compounds (Table 12.2). The Rh–Rh distance of 2.960(1) Å for the Rh2(µ-O2CCF3)2(CO)4 unit is longer than that of 2.984(1) Å found for the dirhodium moieties in the infinite chain {[Rh2(µ-O2CCF3)2(CO)4]2} ,310 a fact which suggests the absence of bonding interactions. The distance of 2.790(1) Å between a Rh atom of Rh2(O2CCF3)4 and a Rh atom of the neighboring Rh2(µ-O2CCF3)2(CO)4 unit is among the longest ax interactions known. Since each Rh atom of the Rh2(µ-O2CCF3)2(CO)4 moiety is essentially a square planar d8 unit, it has the potential to act as a donor to an adjacent acceptor, which in this case is the Rh atom of a Rh2(O2CCF3)4 dimer. The entire repeat unit {[Rh2(µ-O2CCF3)2(CO)4][Rh2(O2CCF3)4][Rh2(µ-O2CCF3)2(CO)4]} can be considered as being held together in the following manner: there is a Rh–Rh contact of 3.062(1) Å between two adjacent Rh2(µ-O2CCF3)2(CO)4 units,50 which is shorter than that found between Rh2(µ-O2CCF3)2(CO)4 moieties in the chain {[Rh2(µ-O2CCF3)2(CO)4]2} (3.092(1) Å).310 These findings give {Rh2(O2CCF3)4[Rh2(µ-O2CCF3)2(CO)4]2} unique features such as the electron delocalization along the chain.50
12.6. Reactions of Rh24+ Compounds
12.6.1 Oxidation to Rh25+ and Rh26+ species
Although the number of Rh25+ species that have been studied are far fewer than those with Rh24+ cores, the redox chemistry of Rh24+ compounds and their Rh25+ analogs has been the subject of considerable interest. The oxidation of complexes that contain the singly bonded Rh24+ core by one or two electrons gives rise to Rh25+ and Rh26+ species with ground state configurations μ2/4β2β*2/*3 and μ2/4β2β*2/*2 and bond orders of 1.5 and 2, respectively. The Rh25+ compounds that have been structurally characterized are listed in Table 12.9.
Table 12.9. Structural data for mixed valence Rh25+ compounds
Compound |
r (Rh–Rh)a |
r (Rh–Lax) |
Donor |
ref. |
|
(Å) |
(Å) |
atom(s) |
|||
|
|
||||
[Rh2(O2CCH3)4(H2O)2]ClO4·H2O |
2.315(2) |
2.22(1) |
O |
693 |
|
|
2.318(2) |
2.23(1) |
|
|
|
[Rh2(O2CC2H5)4(PPri3)2]SbF6 |
2.510(1) |
2.360(1) |
P |
285 |
|
[Rh2(O2CC2H5)4(PCy3)2]SbF6·2CH2Cl2 |
2.509(1) |
2.375(1) |
P |
285 |
|
cis-(2,2)-[Rh2(HNCOCH3)4(H2O)2]ClO4 |
2.399(1) |
2.281(2) |
O |
713 |
|
[Rh2(ClNCOCH3)4(H2O)2]ClO4·2H2O |
2.403(1) |
2.250(9) |
O |
714 |
|
[Rh2(HNCOCH3)4(theophylline)2]NO3·H2O |
2.426(1) |
2.281(7) |
N |
715 |
|
|
2.428(1) |
2.294(7) |
|
|
|
{[Rh2(HNCOCH3)4](µ2-Cl)} |
2.428(1) |
2.581(1) |
Cl |
717 |
|
{[Rh2(HNCOCH3)4](µ2-Cl)·7H2O} |
2.417(1) |
2.564(1) |
Cl |
716,717 |
|
|
|
2.553(1) |
|
|
|
cis-(2,2)-[Rh2(HNCOCH3)4(µ2-Br)] |
2.430(1) |
2.684(1) |
Br |
716,717 |
|
cis-(2,2)-{[Rh2(HNCOCH3)4](µ2-Br)·3H2O} |
2.427(1) |
2.686(1) |
Br |
717 |
|
|
|
2.674(1) |
|
|
|
cis-(2,2)-{[Rh2(HNCOCH3)4](µ2-I)]} |
2.442(1) |
2.859(1) |
I |
717 |
|
cis-(2,2)-[Rh2(HNCOCH3)4(py)2]BF4 |
2.434(1) |
2.215(6) |
N |
719 |
|
trans-(2,2)-[Rh2(mhp)4]SbCl6·2C2H4Cl2 |
2.359(1) |
b |
b |
720 |
|
|
|
||||
(3,1)-[Rh2(mhq)4(py)]PF6·2C2H4Cl2 |
2.402(1) |
2.111(7) |
N |
404 |
|
(3,1)-[Rh2(hq)4(py)]SbCl6·CH2Cl2 |
2.383c |
2.103c |
N |
404 |
|
(3,1)-[Rh2(hq)4(py)]PF6 |
2.403c |
2.120c |
N |
404 |
|
(3,1)-[Rh2(hq)4(py)]PF6·C6H4Cl2 |
2.396c |
2.116c |
N |
404 |

Rhodium Compounds 541
Chifotides and Dunbar
|
Compound |
r (Rh–Rh)a |
r (Rh–Lax) |
Donor |
ref. |
|
(Å) |
(Å) |
atom(s) |
||
|
|
|
|||
|
(3,1)-[Rh2(hq)4(py)]CF3SO3·2C2H4Cl2 |
2.396(1) |
2.097(4) |
N |
404 |
|
[Rh2(DPhF)4(NCCH3)]ClO4 |
2.466(1) |
2.074(6) |
N |
444 |
|
[Rh2(DTolF)4]ClO4 |
2.447(1) |
b |
b |
285 |
|
|
|
|||
|
[Rh2(DTolF)4(H2O)]O2CCF3 |
2.452(2) |
2.165(2) |
O |
722 |
|
[Rh2(DTolF)4][C(CN)3] |
2.463(4) |
2.07(3) |
N |
468 |
|
Rh2(DTolF)3(δ2-NO3)2 |
2.485(1) |
2.09(1) |
O |
460 |
|
|
|
2.38(1) |
|
|
|
cis-[Rh2(DTolF)2(µ-O2CCF3)2(O2CCF3)(AgO2CCF3)2]2d |
2.448(2) |
2.20(1) |
O |
723 |
|
|
|
2.24(2) |
|
|
|
[Rh2(DAniF)4Cl]·CHCl3 |
2.467(1) |
2.400(2) |
Cl |
500 |
|
(4,0)-Rh2(ap)4Cl |
2.406(1) |
2.421(3) |
Cl |
493,494 |
|
(4,0)-[Rh2(ap)4(C>CH)]·CH2Cl2 |
2.439(1) |
2.02(1) |
C |
730 |
|
(4,0)-[(ap)4Rh2(C>C)2Si(CH3)3]·½C6H14 |
2.443(1) |
2.028(7) |
C |
475 |
|
(ap)4Rh2(C>C)2Rh2(ap)4 |
e |
e |
C |
475 |
|
|
|
|||
|
(4,0)-[Rh2(2-Fap)4Cl]·2CH2Cl2 |
2.413(1) |
2.431(3) |
Cl |
732 |
|
(4,0)-[Rh2(2,6-F2ap)4Cl]·2CH2Cl2 |
2.416(1) |
2.465(2) |
Cl |
732 |
|
(4,0)-Rh2(2,4,6-F3ap)4(C>C)2Si(CH3)3 |
2.460(1) |
2.00(1) |
C |
732 |
|
(3,1)-[Rh2(2,6-F2ap)4Cl]·2CH2Cl2 |
2.420(1) |
2.445(1) |
Cl |
732 |
|
(3,1)-[Rh2(F5ap)4Cl]·2CH2Cl2 |
2.415(1) |
2.438(1) |
Cl |
732 |
|
(3,1)-[Rh2(2,6-F2ap)4CN]·2CH2Cl2 |
2.447(1) |
2.031(5) |
C |
732 |
a |
Distances are given with up to 3 decimal digits. |
|
|
|
|
b No ax ligand. |
|
|
|
|
|
c |
Esds not reported. |
|
|
|
|
d ‘Dimer of dimers’ axially linked by CF3CO2 anions. |
|
|
|
|
|
e |
No bond lengths determined due to disorder. |
|
|
|
|
An early study describes the electrochemical oxidation of Rh2(O2CCH3)4 to the stable cation [Rh2(O2CCH3)4]+;679 the electron self-exchange rate constant of [Rh2(O2CCH3)4(D2O)2]0/+ in aqueous media has been determined.680 Chemical oxidation of Rh2(O2CCH3)4 with Br2 and conc. HNO3 affords Rh2(O2CCH3)4Br and Rh2(O2CCH3)4NO3, respectively.681,682 Various electron-transfer reactions involving the [Rh2(O2CCH3)4]+/0 couple have been performed in aqueous683-686 and acetonitrile687 solutions. Electrolytically generated solutions of the paramagnetic Rh25+ carboxylate species with various R groups have been the subject of detailed analyses by EPR, electronic absorption and Raman spectroscopies.688-690
Chemical oxidation of Rh2(O2CCH3)4 with Ce(IV) followed by elution of the crude product from cation exchange resins with 2 M HClO4 leads to isolation691,692 of [Rh2(O2CCH3)4(H2O)2]- ClO4·H2O, which has been the subject of single crystal X-ray studies.693 The structure of the cation is very similar to that of Rh2(O2CCH3)4(H2O)2223 (Fig. 12.1), with the exception of the Rh–Rh bond distance which is shorter by c. 0.07 Å compared to the neutral molecule. This is attributable to the loss of an electron from a /* orbital upon oxidation; SCF-X_-SW calculations have been performed to account for the observed difference.694 The Rh–Rh distance of 2.315(2) Å in [Rh2(O2CCH3)4(H2O)2]ClO4 is the shortest known distance between two rhodium atoms (Tables 12.1-12.9). The compound [Rh2(O2CCH3)4(DMSO)2]ClO4 with O-bound DMSO has been prepared and spectroscopically studied.695
Electrochemical studies on Rh2(O2CR)4L2 compounds indicate that the ease of the Rh24+ core oxidation depends on the nature of both the R group696-698 and the ax ligands.136,408,697,699-701 It has been nicely shown that a linear free energy relationship exists between the E1/2(ox)1 and

542Multiple Bonds Between Metal Atoms Chapter 12
the Hammett constant μ of the R group.696 The effect of the R group on the values of E1/2(ox) can be assessed from the oxidation potentials +0.56 V, +0.65 V and +0.99 V vs Ag/AgCl
of the monothiocarboxylate compounds Rh2(OSCR)4(PPh3)2, for R = CMe3, CH3 and Ph, respectively.125 Likewise, oxidation of Rh2(O2CR)4 becomes more difficult upon substitution of R = CH3 with CF3 (Table 12.10) due to the electron-withdrawing effect of the latter group.696 In Rh2(O2CCF3)4, the strong electron-withdrawing CF3CO2− ligands lower the energy of the highest occupied molecular orbital (HOMO), which is directly related to the E1/2(ox) of the solvated dirhodium species. In the same vein, an increased donating ability of the ax ligand or solvent renders the oxidation process more favorable; a range of c. 0.60 V is spanned by the potentials measured for Rh2(O2CC3H7)4 with various oxygen, nitrogen, sulfur and phosphorus ligands.696 In addition to exhibiting a single-electron oxidation, Rh2(O2CR)4 complexes undergo an irreversible reduction to [Rh2(O2CR)4]−, a species which is not stable but is immediately reduced by one or more electrons to afford a stable mononuclear Rh(I) complex or a reduced dinuclear species.696 EPR spectra have been obtained for both cation and anion radical species of tetracarboxylate compounds.702,703 The Rh26+ compound formulated as Rh2(µ- O2CCH3)2(OH)2(δ1-O2CCH3)2(NH3)2 has been spectroscopically characterized.704 The half-wave oxidation and reduction potentials of the compounds formulated as Rh2(O2CR)2(bpy)2(H2O)2 bear a linear relationship to the dissociation constant of the parent RCO2H acid.705 The species [Rh2(PhCHOHCO2)2(phen)2(H2O)2]2+ catalyzes the electrochemical reduction of CO2.706
Table 12.10. Half wave potentials (V vs SCE) of various dirhodium compounds in CH3CN
Compound |
E1/2(ox)1 |
E1/2(ox)2 |
E1/2(red) |
ref. |
Rh2(O2CCH3)4 |
+1.17 |
|
|
407 |
Rh2(O2CCH3)4 |
+1.3a |
|
|
409 |
Rh2(O2CCF3)4 |
+1.8a |
|
|
696 |
Rh2(µ-O2CCH3)3(µ-HNCOCH3) |
+0.91 |
|
|
407 |
Rh2(µ-O2CCH3)2(µ-HNCOCH3)2 |
+0.62 |
|
|
407 |
Rh2(µ-O2CCH3)(µ-HNCOCH3)3 |
+0.37 |
+1.65 |
|
407 |
Rh2(HNCOCH3)4 |
+0.15 |
+1.41 |
|
407 |
Rh2(HNCOCF3)4 |
+1.09 |
|
|
709 |
Rh2(µ-O2CCH3)3(µ-PhNCOCH3) |
+1.13a |
|
|
678 |
Rh2(µ-O2CCH3)2(µ-PhNCOCH3)2 |
+0.97a |
|
|
678 |
Rh2(µ-O2CCH3)(µ-PhNCOCH3)3 |
+0.76a |
+1.75 |
|
678 |
Rh2(PhNCOCH3)4 |
+0.55a |
+1.65a |
|
710 |
Rh2(PhNCOCH3)4 |
+0.34 |
+1.54 |
|
710 |
Rh2(pyro)4 |
+0.15 |
+1.33 |
|
430 |
Rh2(vall)4 |
+0.04 |
+1.30 |
|
430 |
Rh2(cap)4 |
+0.011b |
|
<0.84 |
1,712 |
Rh2(DPhF)4 |
+0.34a |
+1.15a |
<1.21a |
444 |
Rh2(DPhBz)4 |
+0.23a |
+1.24a |
<1.58a |
447 |
Rh2(DTolF)4 |
+0.25a |
+1.06a |
<1.33a |
446 |
Rh2(DTolF)4 |
<0.23c |
+0.58c |
<1.81c |
446 |
Rh2(DTolF)2(O2CCF3)2(H2O)2 |
+0.52 |
+1.36 |
|
445 |
Rh2(DTolF)2(O2CCF3)2(H2O)2 |
+0.76a |
+1.44a |
|
445 |
a In CH2Cl2.
b The potential was measured vs Ag/AgCl in CH3CN and is reported on the SCE scale by subtracting 0.044 V.
c Half wave potentials (V vs ferrocenium/ferrocene couple) measured in CH3CN.

Rhodium Compounds 543
Chifotides and Dunbar
Oxidation of Rh2(O2CC2H5)4(PR3)2 (R = Cy, Pri) with ferrocenium hexafluoroantimonate affords [Rh2(O2CC2H5)4(PCy3)2]SbF6 and [Rh2(O2CC2H5)4(PPri3)2]SbF6, which have been structurally characterized.285 Interestingly, the Rh–Rh bond distances are by 0.05 Å longer and the Rh–P distances are by 0.12 Å shorter than the respective ones in the corresponding neutral precursors (Tables 12.1 and 12.9). These changes were anticipated by X_-SW calculations performed on Rh2(O2CCH)4(PH3)2301 and EPR studies on Rh2(O2CR)4(PY3)2 (R = Et, CF3; PY3 = PPh3, P(OPh)3, and the cyclic phosphite P(OCH2)3CEt),707 and were later confirmed by DFT calculations for Rh2(O2CEt)4(PR3)2.285 The results are attributed to removal upon oxidation of an electron from the Rh–Rh μ orbital, which is primarily responsible for the lengthening of the Rh–Rh bond and, which becomes the HOMO in dirhodium adducts with ax phosphine molecules due to the strong antibonding interaction with the phosphine μ-donor orbitals.301 Raman spectra have been performed on oxidized dirhodium compounds with vari-
able R groups and the ιRhRh frequency for each case has been identified.708
The class of carboxamidate (12.5) compounds Rh2(O2CR)n(R'NCOR)4-n (R = CH3 or CF3; R' = H or Ph; n = 0-3) exhibits rich electrochemical properties due to the increased electron density on the Rh(II) centers as n increases. In the case of Rh2(O2CCH3)n(HNCOCH3)4−n, the progressive replacement of acetate ligands results in a more accessible one-electron oxidation, and for n = 3 and 4, the appearance of a second one-electron oxidation (Table 12.10).407,408 Both oxidations of Rh2(HNCOCH3)4 are reversible in CH3CN and occur at E1/2 = +0.15 V and +1.41 V.407 Similar reversible diffusion-controlled oxidations are observed in DMSO (E1/2 = +0.31 V) and pyridine (E1/2 = +0.080 V), but, in both solvents, the second oxidation is obscured by
the oxidation limit of the solvent.408 Similarly to Rh2(O2CCH3)4, the values of E1/2(ox) for Rh2(O2CCH3)n(HNCOCH3)4-n are markedly dependent on the presence of ax ligands.408,423,424
As expected, the substitution of CH3 for CF3 in the acetamidate complexes results in the singleelectron oxidation of Rh2(HNCOCF3)4 being less accessible than that of Rh2(HNCOCH3)4. In a variety of nonaqueous solvents, a one-electron oxidation of Rh2(HNCOCF3)4 is observed at potentials between +0.91 V and +1.09 V vs SCE.709 The mixed acetate/N-phenylacetamidate complexes Rh2(O2CCH3)n(PhNCOCH3)4-n, n = 0-3, have been studied by cyclic voltammetry and, similarly to their acetate/acetamidate analogs, they show a steady decrease in the E1/2(ox) values as the number of N-phenylacetamidate ligands increases;409 for n = 0, 1, the compounds exhibit two reversible one-electron oxidation steps (Table 12.10).710 The oxidation potential of the caprolactamate (cap; anion of 1-aza-2-cycloheptanone) complex Rh2(cap)4711 is the least positive among all the carboxamidates (Table 12.10), thus Rh2(cap)4 is the most electron-rich member of the dirhodium carboxamidate series.1,712
Due to the low oxidation potential of Rh2(HNCOCH3)4, it can be easily oxidized with 30-35% H2O2, in the presence of small amounts of HClO4 or HNO3, to afford [Rh2(HNCOCH3)4(H2O)2]ClO4.713 The structural determination of [Rh2(HNCOCH3)4(H2O)2]- ClO4 revealed the preferred cis-(2,2) arrangement (Fig. 12.20a) and a Rh–Rh bond distance of 2.399(1) Å.713 This distance is longer by 0.08 Å than the Rh–Rh distance of the Rh25+ tetraacetate adduct [Rh2(O2CCH3)4(H2O)2]ClO4693 and shorter by 0.016 Å than the Rh–Rh distance of the Rh24+ precursor cis-(2,2)-[Rh2(HNCOCH3)4(H2O)2].414 The longer Rh–Rh bond distance compared to the tetraacetate adduct is attributed to steric factors,713 whereas the shorter Rh–Rh distance compared to its neutral Rh24+ precursor is expected due to the higher bond order of [Rh2(HNCOCH3)4(H2O)2]ClO4. The crystal structure determination of the chlorinated analog [Rh2(ClNCOCH3)4(H2O)2]ClO4 reveals a similar cis-(2,2) arrangement to [Rh2(HNCOCH3)4- (H2O)2]ClO4 and essentially identical Rh–Rh bond distances (2.40 Å).714 Oxidation of the reaction product between Rh2(HNCOCH3)4 and theophylline with HNO3 affords the reddish-
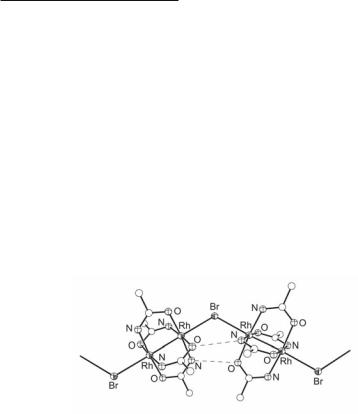
544Multiple Bonds Between Metal Atoms Chapter 12
violet mixed-valence paramagnetic compound [Rh2(HNCOCH3)4(theophylline)2]NO3 which has been structurally characterized (Section 12.7.3).715
The [Rh2(HNCOCH3)4]+ units have been successfully linked by bridging halide ions to form a series of infinite zig-zag chains of general formulae {[Rh2(HNCOCH3)4](µ2-X)} (X = Cl, Br, I), which have been structurally characterized (Fig. 12.36).716,717 These infinite chains are mutually parallel in the crystal and represent the first such example of paddlewheel-type Rh25+ compounds. The chains are supported by hydrogen bonds between the NH and O groups of adjacent acetamidate bridging groups and occasionally by interstitial water molecules. The increase in the Rh–Rh bond length from the chloride to the iodide bridged complex (Table 12.9) is in accordance with the increasing μ-donating ability of the ax halide ions, Cl < Br < I.717 The Rh–Rh bond distances (2.427(1)-2.442(1) Å) in {[Rh2(HNCOCH3)4](µ2-X)} (X = Cl, Br, I) are longer than that in [Rh2(HNCOCH3)4(H2O)2]+ (2.399 Å). The magnetic interactions along the chains of these compounds have been studied.716-718 Polymeric complexes of acetamidate dimers linked by bidentate ligands have been prepared and magnetic measurements of their Rh25+ analogs have been reported.719
Fig. 12.36. The structure of the infinite zig-zag chain {[Rh2(µ-HNCOCH3)4](µ2-Br)} .
The crystal structure determination of the paramagnetic compound trans-(2,2)-[Rh2(mhp)4]- SbCl6·2C2H4Cl2 revealed overlap of the neighboring aromatic rings and a Rh–Rh bond distance of 2.359(1) Å, 720 which is essentially the same as that of the neutral precursor396,397 (Table 12.3). The lack of change in the Rh–Rh bond distance in the oxidized species is attributed to the fact that the antibonding character of the β*(Rh–Rh*) orbital, from which the electron is removed, is not always sufficient to overcome the increased repulsion caused by the increase of the positive charge of the metal atoms.720 The non-systematic changes of the Rh–Rh bond lengths among the different salts of the (3,1)-[Rh2(hq)4(py)]+ ion (Table 12.9), which also has a β*(Rh–Rh*) HOMO orbital compared to the neutral precursor, are accounted for by the foregoing argument.404
In contrast to dirhodium compounds supported by carboxylate ligands, which undergo only a single oxidation, complexes supported by tetraamidinate bridging groups (12.7) primarily undergo two metal centered one-electron oxidations. The latter correspond to stepwise removal of electrons from the β*(Rh–Rh*) orbital (HOMO),442,721 owing to the presence of the more basic amidinate groups which make the compounds more electron-rich. The electrochemical properties of Rh2(DArF)4 (DArF = DPhF, DTolF), Rh2(DPhBz)4 and the EPR spectra of the corresponding paramagnetic cations have been studied.444,446,447 The previous compounds display reduction processes to Rh23+ species by addition of an electron to the μ*(Rh–Rh) orbital (LUMO)444,446,447 but, in the case of Rh2(DTolF)4, the reduction process is irreversible.446 A systematic study of the series Rh2(ArNCHNAr)4, (Ar = XC6H4, X = p-OMe, p-Me, H, m-OMe, p-Cl, m-Cl, m-CF3, p-CF3, or Ar = 3,4-Cl2C6H3) revealed that the electrode potentials of the