
Multiple Bonds Between Metal Atoms / 12-Rhodium Compounds
.pdf
Rhodium Compounds 545
Chifotides and Dunbar
compounds are linearly related to the Hammett constant μ of X and that both the E1/2(ox) and E1/2(red) potentials become more positive as the electron-withdrawing ability of the aryl substituent increases.442 Therefore, electron-withdrawing and electron-donating substituents facilitate the corresponding reduction and oxidation processes occurring at the dirhodium core.442 In contrast to Rh2(DPhF)4, which exhibits a single reduction at E1/2 = -1.21 V (vs SCE) in CH3CN, Rh2(DPhF)4(CNPh) and (DPhF)4Rh2(CNPhNC)Rh2(DPhF)4 do not exhibit a reduction within the negative potential limit of the solvent (−1.8 V). This result suggests that the LUMOs of the latter compounds are higher in energy than the LUMO of Rh2(DPhF)4; this is accounted for by the μ-donation of electron density from the ax ligands CNC6H5 and CNPhNC to the antibonding orbitals of the dimetal core.475
Bulk electrolysis of Rh2(DPhF)4 at +0.65 V in the presence of But4NClO4 affords [Rh2(DPhF)4(NCCH3)]ClO4 which has been structurally characterized.444 The Rh–Rh bond length of 2.466(1) Å is slightly longer than the Rh–Rh bond in the neutral precursor Rh2(DPhF)4(NCCH3) (2.459(1) Å) and the complex Rh2(DPhF)4 with no ax ligands (2.457(1) Å). The Rh–Rh bond distances in [Rh2(DTolF)4]ClO4285 and Rh2(DAniF)4Cl500 are longer by c. 0.015(1) Å compared to the Rh24+ precursors (Tables 12.4 and 12.9). Likewise, the Rh–Rh bond in [Rh2(DTolF)4(H2O)]O2CCF3 (2.452(2) Å)722 is longer than that in Rh2(DTolF)4 (2.434(1) Å) and [Rh2(DTolF)4]ClO4 (2.447(1) Å) with no ax ligands. It may thus be inferred that, for compounds with formamidinate bridging groups, the Rh–Rh bonds of the oxidized species are lengthened compared to the neutral counterparts, although changes of the Rh–Rh bond lengths are minimal with no apparent trend, when the electron is removed from a β*(Rh–Rh*) orbital (HOMO).285
Correlations of the Rh–Rh bond distances of the Rh24+ precursors with that of the paramagnetic ‘dimer of dimers’ cis-[Rh2(DTolF)2(µ-O2CCF3)2(O2CCF3)(AgO2CCF3)2]2 (2.448(2) Å),723 can not be made due to the different ax ligands involved. The Rh–Rh distance in cis-[Rh2(DTolF)2(µ- O2CCF3)2(O2CCF3)(AgO2CCF3)2]2 is longer than that in cis-Rh2(DTolF)2(O2CCF3)2(H2O)2 (2.425(1) Å), but shorter than that in cis-Rh2(DTolF)2(O2CCF3)2(NCCH3)2 (2.474(5) Å). The single-electron oxidation of cis-Rh2(DTolF)2(O2CCF3)2(H2O)2 affords the blue colored species [Rh2(DTolF)2(O2CCF3)2]+,445 which has been isolated in the solid state as the paramagnetic complexes [Rh2(DTolF)2(O2CCF3)2(H2O)2]ClO4 and Rh2(DTolF)2(O2CCF3)(NO3)2.723 Analytical data show that the oxidation of the Rh24+ precursor proceeds with elimination of a trifluoroacetate group, therefore Rh2(DTolF)2(O2CCF3)(NO3)2 most likely contains a bidentate nitrate group coordinated to each Rh center. Such a binding mode has been observed for the paramagnetic compound Rh2(DTolF)3(δ2-NO3)2 which has been structurally characterized.460 The rather long Rh–Rh bond in Rh2(DTolF)3(δ2-NO3)2 (2.485(1) Å) is attributed to the reduced number of bridging ligands.460 The second oxidation of cis-Rh2(DTolF)2(O2CCF3)2(H2O)2 generates the Rh26+ species which is not stable, but undergoes changes that lead to further oxidizable products.445 It is notable that the Rh24+-porphyrin-based molecular boxes,469,470 as well as the molecular triangle and square assembled from Rh24+ formamidinate units with oxalate linkers (Section 12.7.2), are multiredox systems with distinctly different electrochemical behavior depending on the structure of the compound, e.g., the second oxidation waves for the Rh2 oxalate square and triangle are at 845 and 1125 mV, respectively.13
Reaction of Rh2(DTolF)4 with X = TCNE, TCNQ and DM-DCNQI proceeds via a single electron transfer from the dimetal unit to the cyano ligand to afford Rh2(DTolF)4X which contains the [Rh2(DTolF)4]+ cation radical and the cyano ligand as a radical anion.468 Electrochemical and EPR measurements suggest a different extent of coordination between the polycyano fragment and the dirhodium unit, depending on the polarity of the solvents. Unexpectedly, for the TCNE complex, the tetracyanoethylenide ion is transformed to the tricyanomethanide

546Multiple Bonds Between Metal Atoms Chapter 12
anion [C(CN)3]−, thus affording crystals of [Rh2(DTolF)4][C(CN)3], as shown by X-ray crystallography.468
The paramagnetic compound Rh2(DTolF)4(C>CC5H4N) has been synthesized and used as a starting material to prepare heterotrimetallic Rh/Re complexes with 4-ethynylpyridine as a bridging moiety;724 the interactions between the Rh25+ and Re units, however, are weak as indicated by CV and EPR experiments.
The crystal structures and the redox properties of a number of Rh25+ complexes with the anilinopyridinate ligand 12.10 (ap) have been reported. The compound Rh2(ap)4 undergoes two accessible single-electron oxidations to the corresponding Rh25+ and Rh26+ species which have been spectroscopically characterized;490-493 the latter dication is postulated to possess a Rh–Rh double bond.491 The symmetric isomer trans-(2,2)-Rh2(ap)4 undergoes two single-elec- tron oxidation processes at E1/2 = +0.01 and +0.64 V vs Ag/AgCl.490 The Rh25+ compound (4,0)-Rh2(ap)4Cl, which has been crystallographically characterized,493,494 is prepared by reaction of Rh2(O2CCH3)4 with molten 2-anilinopyridine followed by dissolution in a CH2Cl2/CCl4 mixture and separation on a silica gel column. The chloride ion source appears to be the solvent.493 It has been reported that Rh2(ap)4Cl can be prepared by refluxing RhCl3·3H2O and ap in anhydrous EtOH.725 The compound Rh2(ap)4Cl is reversibly reduced by one electron at -0.38 V and reversibly oxidized at +0.52 V vs SCE in CH2Cl2. The singly oxidized form of Rh2(ap)4 and the cation of Rh2(ap)4Cl exhibit different UV and EPR spectra and therefore different distributions of the odd-electron density; the EPR spectra of Rh2(ap)4 and Rh2(ap)4Cl are consistent with a singly occupied molecular orbital (SOMO) being equally distributed on both Rh atoms in the former complex and localized on one rhodium atom in the latter, respectively.493 The (4,0)726,727 and trans-(2,2)727,728 isomers of Rh2(ap)4 react rapidly with dioxygen to form the stable superoxide complex Rh2(ap)4(O2) wherein molecular oxygen is axially bound to the dirhodium unit. Moreover, the compound Rh2(ap)4Cl reduces dioxygen on a pure carbon paste electrode generating H2O2, which can be accurately measured.729 The reaction of NaR
or LiR (R = C>CH−, C>CPh−, C>CC5H11− or C>C(CH2)4C>CH−) with the chloride complex Rh2(ap)4Cl in THF affords the paramagnetic compounds Rh2(ap)4R with Rh–C μ-bonds.730
These complexes have been characterized by EPR, infrared, electronic absorption and mass spectroscopies and, in the case of Rh2(ap)4(C>CH), by X-ray crystallography.730 The increase in the Rh–Rh bond distance from 2.406(1) Å in Rh2(ap)4Cl to 2.439(1) Å in Rh2(ap)4(C>CH) reflects the strong electron-donating properties of C>CH-. Electrochemical studies have shown that both the E1/2(ox) and E1/2(red) values for Rh2(ap)4R are shifted cathodically from the potentials of the parent compound Rh2(ap)4Cl; the second oxidations are observed at c. +1.0 V. These
changes reflect an increase in the electron density of the Rh(Naniline)4 atom upon ax binding of the group R.730 Disproportionation of Rh2(ap)4X (X = Cl, Br), in the presence of acids, affords
protonated Rh22+ dimers.731
A series of Rh25+ compounds of the type Rh2L4Cl (L: (2-fluoroanilino)pyridinate: 2-Fap; (2,6-difluoroanilino)pyridinate: 2,6-F2ap; (2,4,6-trifluoroanilino)pyridinate: 2,4,6-F3ap; (2,3,4,5,6-pentafluoroanilino)pyridinate: F5ap) have been reported.732 The compound Rh2(2- Fap)4Cl exists only as the (4,0) isomer, whereas Rh2(2,6-F2ap)4Cl, Rh2(2,4,6-F3ap)Cl and Rh2(F5ap)4Cl exist as both (4,0) and (3,1) isomers with a preference for the (3,1) structure. The EPR and magnetic data indicate that the SOMO is a β*(Rh–Rh*) orbital and that the electronic configuration of these compounds is μ2/4β2/*4β*1.732 The compounds with 2,6-F2ap and 2,4,6-F3ap ligands undergo one reversible single-electron reduction and two reversible single-electron oxidations. The electronic effect of the substituents for these compounds can be quantified by a linear relationship between the E1/2 values and the Hammett parameter μ of the bridging ligand substituents.732 The preparation, electrochemistry and spectroscopic

Rhodium Compounds 547
Chifotides and Dunbar
properties of (ap)4Rh2(C>C)2Si(CH3)3 and (ap)4Rh2(C>C)2Rh2(ap)4 have been reported, and the structure of (ap)4Rh2(C>C)2Si(CH3)3 has been determined.475 Both compounds exhibit redox processes associated with reduction to the species with Rh24+ units and oxidation to the species with Rh26+ units. For (ap)4Rh2(C>C)2Rh2(ap)4, the two reduction processes are separated by 130 mV, which is an indication of electronic interaction between the dirhodium units.475
Oxidation of Na4[Rh2(SO4)4(H2O)2]·5H2O with Ce(IV) in sulfuric acid followed by cation exchange leads to the paramagnetic compounds M3[Rh2(SO4)4(H2O)]·nH2O (M = K, n = 3; M = Cs, n = 1), which have been studied by EPR and electronic spectroscopies.733 Likewise, oxidation of Na4[Rh2(CO3)4(H2O)2]·4H2O in acetonitrile with (NH4)2[Ce(NO3)6] has been purported to afford Na3[Rh2(CO3)4(H2O)2]·2H2O.366
12.6.2 Cleavage of the Rh–Rh bond
A wide range of reagents and reaction conditions can lead to cleavage of the Rh–Rh bond. Reaction of Rh2(O2CCH3)4 with ArSO2H affords Rh26+ compounds,734 whereas the hydrohalic acids HCl and HBr, as gases or in acetone or ethanol solutions, induce decomposition of Rh2(O2CCH3)4 to RhX3 (X = Cl, Br) and rhodium metal.735,736 Dirhodium tetraacetate is stable towards O2, but is converted by O3 to the [Rh3(µ3-O)(µ-O2CCH3)6(H2O)3]+ ion.737,738 As stated in Section 12.4.1, reaction of Rh24+(aq) with O2 in a 2-3 M HClO4 solution is postulated to produce the purple paramagnetic superoxo complex [Rh2(O2−)(OH)2(H2O)n]3+.593,594 Reactions of [Rh2(H2O)10]4+ in the presence of Cl-, Br- or I- ions lead to formation of Rh metal.739 Carbon monoxide forms a labile adduct with Rh2(O2CCH3)4, which is isolated only at low temperatures,261 whereas Rh6(CO)16 is formed at very high pressures of CO (300 atm) in C4H9OH above 120 ºC.262 Phosphines and phosphites readily react with dirhodium compounds to form products other than merely ax adducts. Thus, PPh3 reacts with a solution of Rh2(O2CCH3)4 in MeOH/HBF4 to afford Rh(PPh3)3BF431,291 and reaction with excess of the bicyclic phosphite 4-ethyl-2,6,7-trioxa-1-phosphabicyclo[2.2.2]octane results in production of a mononuclear Rh(I) species.281 Accordingly, reactions of Rh2(O2CCF3)4 with PMe2Ph, PPh3 and P(OMe)3 lead to cleavage of the Rh–Rh bond and production of mononuclear Rh(I) and Rh(III) compounds.142,290 In the presence of PMe3, interaction between dialkylor diarylmagnesium and Rh2(O2CCH3)4 affords mononuclear Rh(I) compounds.740,741 Reaction of cis-[Rh2(O2CCH3)2(NCCH3)6](BF4)2 with PCy3 leads to cleavage of the Rh–Rh bond and formation of [Rh(CH3CN)2(PCy3)2]BF4,742 whereas reaction of [Rh2(NCCH3)10](BF4)4 with (diphenylphosphino)tetrathiafulvalene affords mononuclear Rh(I) compounds.743 Reaction of Rh2(O2CCH3)4 with dppm in the presence of Me3SiCl affords RhCl3(dppm)(NCCH3) in low yield744 (the main product is Rh2(µ-dppm)2Cl4; Section 12.3.5).507 Electrolysis of Rh2(HNCOCH3)4(SbPh3)2 in CH2Cl2, in the presence of excess SbPh3, produces the monomeric Rh(III) compound Rh(Ph)(SbPh3)2Cl2(NCCH3).745
12.7 Applications of Dirhodium Compounds
12.7.1 Catalysis
Dirhodium tetracarboxylate compounds catalyze many reactions including asymmetric cyclopropanation and cyclopropenation, carbon–hydrogen insertion and carbenoid initiated C–C bond formation.1-5 The catalytically active dirhodium compounds bearing orthometalated phosphine and homochiral carboxamidate groups that have been structurally characterized are listed in Tables 12.2 and 12.3, respectively. Further coverage of this topic is presented in Chapter 13.
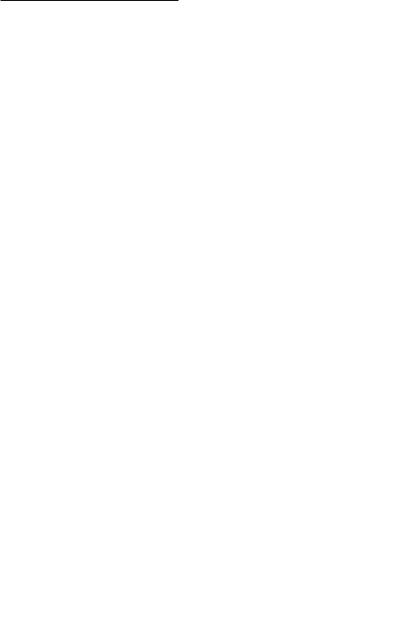
548Multiple Bonds Between Metal Atoms Chapter 12
12.7.2 Supramolecular arrays based on dirhodium building blocks
A pioneering contribution in promoting the assembly and dictating the main structural features of supramolecular arrays is the introduction of subunit precursors with Rh–Rh bonds linked by various eq and ax polyfunctional bridging groups such as polycarboxylate anions (12.48-12.56), polypyridyls (12.57-12.58), and polynitriles (12.59-12.60).13,15 The precursors of choice have been Rh2(cis-formamidinate)22+ moieties (typically DAniF; 12.7) with two cisoid, non-labile formamidinate anions. The dirhodium core with dicarboxylic acids has also been successfully employed as a substitutionally inert corner piece.111,114 The remaining eq positions are typically occupied by labile ligands such as carboxylate or acetonitrile groups which are easily replaced by polyfunctional linkers (e.g., dicarboxylates). The strong ax interactions that the Rh24+ units usually engage in, renders them suitable candidates for linking by means of eq and ax connectors. This combination of both types of linking modes (Fig. 12.37) allows the formation of 1- and 2D molecular tubes, loops, squares, triangles, helices and other supramolecular arrays.13,15 The judicious choice of both eq and ax elements provides fine control over the nature and degree of interaction between adjacent dimetal units and an enormous diversity in the emerging structures. The resulting oligomers and networks are neutral rather than highly charged species that retain their structural integrity upon oxidation in a controlled fashion. A wide variety of organic ligands may be used to vary the electrochemical behavior, solubility as well as the magnetic and spectroscopic properties. The supramolecular arrays function as hosts for medium size guest molecules, and the sizes of the pores, interstices and channels can be tuned to serve as sequestration and separation reagents. The dirhodium building blocks and the emerging supramolecular arrays that have been crystallographically determined are listed in Tables 12.1, 12.4 and 12.5.
Fig. 12.37. Three basic modes of assembly of dirhodium units.
An example of a neutral Rh24+ molecular triangle is that of [Rh2(cis-DAniF)2(µ2-C2O4)]3 (Fig. 12.38).478 The molecular triangle is formed exclusively by using 1 equiv of the oxalate anion 12.48 per equiv of [Rh2(cis-DAniF)2(NCCH3)4](BF4)2, whereas the use of an excess of oxalate anions leads exclusively to the molecular square [Rh2(cis-DAniF)2(µ2-C2O4)]413,478 (Fig. 12.39). The distinct electrochemical signatures of the square and the triangle (the second and third oxidation processes take place at much higher potentials for the triangle) have allowed the study of the equilibrium between these species in solution (see Section 12.6.1).13
Among systems built from dimetal units, the present one is unique in that the same linker
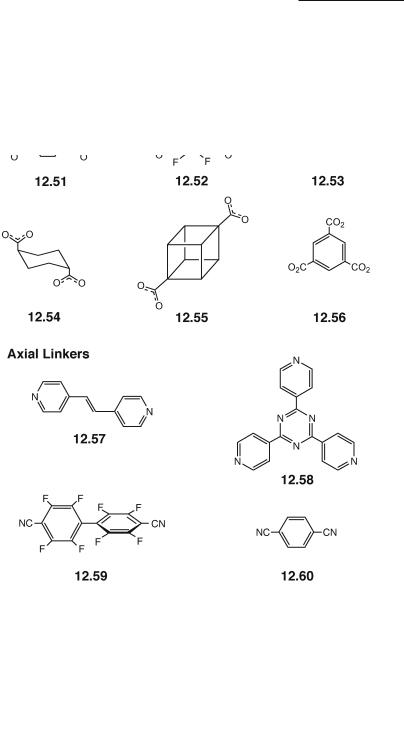
Rhodium Compounds 549
Chifotides and Dunbar
forms both a triangle and a square; the isolation of a particular structural motif is subject to kinetic as well as thermodynamic control. The square and the triangle have significantly different gel-permeation chromatography retention times and artificial mixtures of them have been successfully separated by this technique.746 An interesting application of the redox properties of the square and the triangle is their use as potential switches to turn on and off their affinity for anions; in the oxidized (cationic) state, they could readily entrap suitably sized anions and in their reduced or neutral state, disgorge them.
In contrast to the previous case where the formation of triangles or squares from the combination of [Rh2(cis-DAniF)2]2+ units with oxalate anions depends on the reaction conditions,
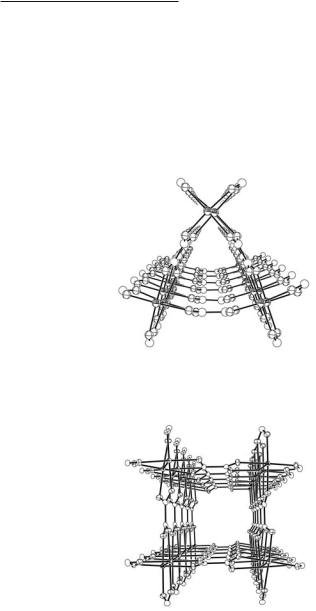
550Multiple Bonds Between Metal Atoms Chapter 12
only molecular triangles are formed when the orthometalated units {Rh2[Ph2P(C6H4)]2}2+ react with rigid linear dicarboxylate groups (e.g., eq linkers 12.48 and 12.51).564 This is partially due to the fact that the corner piece {Rh2[Ph2P(C6H4)]2}2+ has a preferred twist of c. 23° about the Rh–Rh bond (there is no inherent resistance to moderate twisting about the Rh–Rh axis, since there is only a net μ-bond), resulting in a very small strain in the triangular relative to the square structure; this allows the thermodynamic factor favoring the smaller ring (entropy) control the outcome of the reaction.564 A number of these molecular triangles such as {Rh2[Ph2P(C6H4)]2(µ2- C2O4)(py)2}3 (Fig. 12.40) have been structurally characterized (Table 12.5).564,747
Fig. 12.38. Stacking arrangement for the Rh2 molecular triangles [Rh2(cis-DAniF)2(µ2-C2O4)]3.
Fig. 12.39. Stacking arrangement for the Rh2 molecular squares [Rh2(cis-DAniF)2(µ2-C2O4)]4. The anisyl groups have been omitted for the sake of clarity.
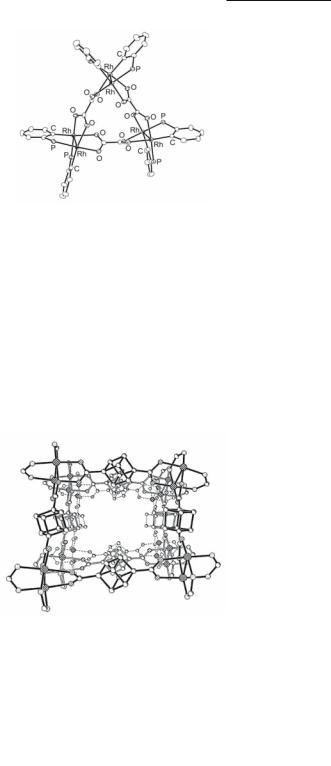
Rhodium Compounds 551
Chifotides and Dunbar
Fig. 12.40. The molecular triangle {Rh2[Ph2P(C6H4)]2(µ2-C2O4)(py)2}3. The phenyl groups and the ax py ligands have been omitted for the sake of clarity.
A series of molecular squares of composition [Rh2(DAniF)2(CH3CNax)2(O2CXCO2)]4 (X = spacer group), have been prepared by reacting [Rh2(cis-DAniF)2(NCCH3)4](BF4)2 with each of the dicarboxylate linkers 12.49-12.55 in 1:1 ratio.479,483 The molecular structure of the Rh2 cubanedicarboxylate square is illustrated in Fig. 12.41. In all cases, the packing in the crystals creates infinite channels 12.61 that are capable of accommodating solvent molecules. Similar channels that can entrap small molecules are formed by molecular boxes with chelating dicarboxylate molecules capping the dirhodium units at the corners and substituted benzene-1,4-dicarboxylate side walls (Fig. 12.42).111,114 A survey of the effect of the aromatic substituents on the basic box skeleton showed that substituents larger than the OH group cause distortion of the walls and an overall saddle-like distortion of the framework.114
Fig. 12.41. Core of the Rh2 cubanedicarboxylate square {[Rh2(cis-DAniF)2(CH3CNax)2]- (1,4-cubanedicarboxylate)}4. The anisyl groups and the ax ligands have been omitted for the sake of clarity.
12.61
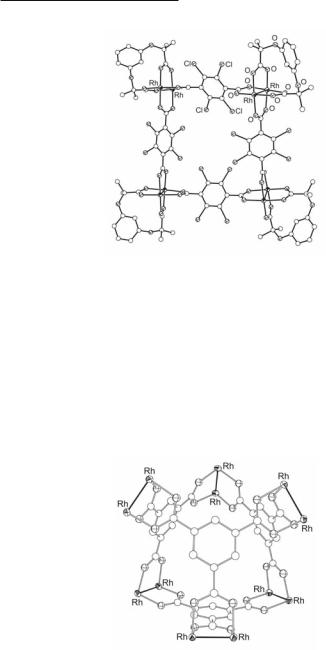
552Multiple Bonds Between Metal Atoms Chapter 12
Fig. 12.42. The molecular square {Rh2[O2C(CH3)2OC6H4OC(CH3)2CO2](µ2-O2CC6Cl4CO2)}4.
A discrete molecular cage formulated as {[Rh2(cis-DAniF)2]6[µ3-C6H3(CO2)3]4(CH3CNax)7.5} (Fig.12.43) has been assembled by reaction of 6 equiv of Rh2(cis-DAniF)2(NCCH3)4](BF4)2 with
4 equiv of the anion of 1,3,5-tricarboxylatobenzene 12.56 (trimesic acid).482 The 1H NMR spectrum of the cage exhibits one resonance for all 12 aromatic protons on the four C6H3(CO2)33− linkers and equivalent resonances for the 12 DAniF anions. The centers of the four six-mem- bered rings define a tetrahedron, and the midpoints of the six Rh24+ units define an octahedron. The overall idealized symmetry is Td and a well-ordered CH2Cl2 molecule is encapsulated in the center of the Rh2 cage. Despite the propensity of dirhodium centers to bind ax ligands, only 2/3 of the ax sites are occupied by CH3CN molecules due to steric crowding.13,482
Fig. 12.43. The core of {[Rh2(cis-DAniF)2]6[µ3-C6H3(CO2)3]4(CH3CNax)7.5}.
Dirhodium-porphyrin-based molecular boxes exhibiting rich photochemical and redox properties have been prepared by reaction of Rh2(DTolF)2(O2CCF3)2 with a variety of functionalized porphyrins.469,470 Depending on the number and position of the peripheral pyridyl469 or carboxylate470 substituents, porphyrins can be employed as linear or angular connectors to form molecular boxes470 12.62 or 2-D layers 12.63 with high porosity,748 but the compounds formed have not been structurally characterized.
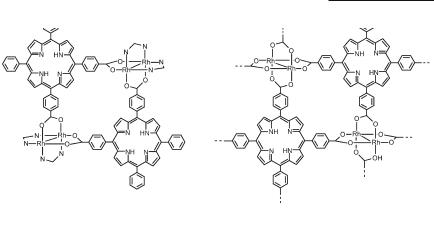
Rhodium Compounds 553
Chifotides and Dunbar
12.62 |
12.63 |
In view of the affinity of Rh24+ units for ax ligands, the combination of ax and eq linkers has been employed to prepare extended 1-, 2- and 3-D arrays based on dirhodium building blocks. By using the Rh2 molecular square [Rh2(cis-DAniF)2(µ2-C2O4)]4 (Fig. 12.44a) with the ax linker 12.59, the compound {[Rh2(cis-DAniF)2]4(µ2-C2O4)4(NCC6F4C6F4CN)4} is formed, exhibiting infinite tubes of square cross section and entrapped molecules of CH2Cl2 within the tube.479 A portion of the extended structure is illustrated in Fig. 12.44b. When the eq linkers of the assembly units Rh2(cis-DAniF)22+ are changed from 12.48 (oxalate anion) to 12.49 (malonate anion), the molecular loop 12.64 is formed.479 Reaction of loop 12.64 with the ax linker trans-1,2-bis(4-pyridyl)ethylene 12.57, results in the formation of the 1-D tubular molecule {[Rh2(cis-DAniF)2]2(µ2-O2CCH2CO2)2(NC5H4CHCHC5H4N)2} (12.65).479 As can be observed from the schematic representation, the loops are related alternately by centers of inversion and two-fold axes in an overall linear structure and, interestingly, there are no guest molecules inside the tubes. Reaction of the loop 12.64 with the ax linker 12.59 affords another 1-D molecular tube {[Rh2(cis-DAniF)2]2(µ2-O2CCH2CO2)2(NCC6F4C6F4CN)2} (12.66) with interstitial CH2Cl2 molecules located inside the tubes. Since the [Rh2(cis-DAniF)2]4(µ2-O2CCH2CO2)]2 loop units impose significant steric demands, in the direction parallel to the metal-metal bond, only longer ax linkers that prevent the close approach of the bulky p-anisyl groups lead to formation of infinite columns. Shorter linkers such as 1,4-dicyanobenzene 12.60, favor 2-D sheetlike structures 12.67, which permit the C6H4OMe groups of the Rh24+ units to avoid steric interactions. As shown schematically, each rectangular sheet belongs to the 2-D group Cmm, which is the highest symmetry possible for such a case. Reaction of the loop 12.64 with the ax linker tri(4-pyridyl)triazine 12.58 in a stepwise fashion, by varying the stoichiometric ratio of the reactants, leads to construction of impressive self-assembled 3-D structures. When the reaction is performed in a 1:2 molar ratio of the Rh24+ loop unit to the linker 12.58, a 1-D zigzag molecular tunnel of composition {[Rh2(cis-DAniF)2]2(µ2-O2CCH2CO2)2[C3N3(C5H4N)3]2} (12.68) is formed, with no solvent molecules enclosed in the tunnel.481 Reaction of the loop
12.64 with the ax linker tri(4-pyridyl)triazine 12.58 in a 3:4 molar ratio reveals that the additional loop is linking two zig-zag tunnels through the open nitrogen coordination sites of the triazine ligands to afford interpenetrating networks; the structure may be described as a collection of double helices formulated as {[Rh2(cis-DAniF)2]2(µ2-O2CCH2CO2)2}3[C3N3(C5H4N)3]4} (12.69), each with a pitch of c. 45° and surrounded by six other double helices.13,15,481
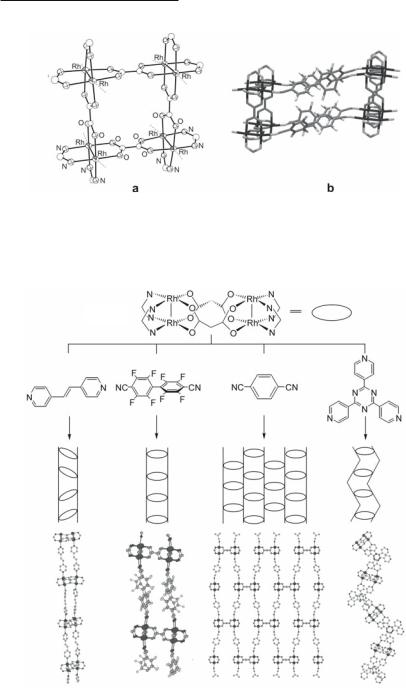
554Multiple Bonds Between Metal Atoms Chapter 12
Fig. 12.44. (a) Molecular square [Rh2(cis-DAniF)2(µ2-C2O4)]4 and (b) portion of the ex-
tended structure of the infinite tubes {[Rh2(cis-DAniF)2]4(µ2-C2O4)4(NCC6F4C6F4CN)4} . The anisyl groups and the entrapped molecules of CH2Cl2 within the tube have been omitted.
12.64
12.65 |
12.66 |
12.67 |
12.68 |