
- •Contents
- •Preface
- •1.1 Elementary thermodynamic ideas of surfaces
- •1.1.1 Thermodynamic potentials and the dividing surface
- •1.1.2 Surface tension and surface energy
- •1.1.3 Surface energy and surface stress
- •1.2 Surface energies and the Wulff theorem
- •1.2.1 General considerations
- •1.2.3 Wulff construction and the forms of small crystals
- •1.3 Thermodynamics versus kinetics
- •1.3.1 Thermodynamics of the vapor pressure
- •1.3.2 The kinetics of crystal growth
- •1.4 Introduction to surface and adsorbate reconstructions
- •1.4.1 Overview
- •1.4.2 General comments and notation
- •1.4.7 Polar semiconductors, such as GaAs(111)
- •1.5 Introduction to surface electronics
- •1.5.3 Surface states and related ideas
- •1.5.4 Surface Brillouin zone
- •1.5.5 Band bending, due to surface states
- •1.5.6 The image force
- •1.5.7 Screening
- •Further reading for chapter 1
- •Problems for chapter 1
- •2.1 Kinetic theory concepts
- •2.1.1 Arrival rate of atoms at a surface
- •2.1.2 The molecular density, n
- •2.2 Vacuum concepts
- •2.2.1 System volumes, leak rates and pumping speeds
- •2.2.2 The idea of conductance
- •2.2.3 Measurement of system pressure
- •2.3 UHV hardware: pumps, tubes, materials and pressure measurement
- •2.3.1 Introduction: sources of information
- •2.3.2 Types of pump
- •2.3.4 Choice of materials
- •2.3.5 Pressure measurement and gas composition
- •2.4.1 Cleaning and sample preparation
- •2.4.3 Sample transfer devices
- •2.4.4 From laboratory experiments to production processes
- •2.5.1 Historical descriptions and recent compilations
- •2.5.2 Thermal evaporation and the uniformity of deposits
- •2.5.3 Molecular beam epitaxy and related methods
- •2.5.4 Sputtering and ion beam assisted deposition
- •2.5.5 Chemical vapor deposition techniques
- •Further reading for chapter 2
- •Problems for chapter 2
- •3.1.1 Surface techniques as scattering experiments
- •3.1.2 Reasons for surface sensitivity
- •3.1.3 Microscopic examination of surfaces
- •3.1.4 Acronyms
- •3.2.1 LEED
- •3.2.2 RHEED and THEED
- •3.3 Inelastic scattering techniques: chemical and electronic state information
- •3.3.1 Electron spectroscopic techniques
- •3.3.2 Photoelectron spectroscopies: XPS and UPS
- •3.3.3 Auger electron spectroscopy: energies and atomic physics
- •3.3.4 AES, XPS and UPS in solids and at surfaces
- •3.4.2 Ratio techniques
- •3.5.1 Scanning electron and Auger microscopy
- •3.5.3 Towards the highest spatial resolution: (a) SEM/STEM
- •Further reading for chapter 3
- •Problems, talks and projects for chapter 3
- •4.2 Statistical physics of adsorption at low coverage
- •4.2.1 General points
- •4.2.2 Localized adsorption: the Langmuir adsorption isotherm
- •4.2.4 Interactions and vibrations in higher density adsorbates
- •4.3 Phase diagrams and phase transitions
- •4.3.1 Adsorption in equilibrium with the gas phase
- •4.3.2 Adsorption out of equilibrium with the gas phase
- •4.4 Physisorption: interatomic forces and lattice dynamical models
- •4.4.1 Thermodynamic information from single surface techniques
- •4.4.2 The crystallography of monolayer solids
- •4.4.3 Melting in two dimensions
- •4.4.4 Construction and understanding of phase diagrams
- •4.5 Chemisorption: quantum mechanical models and chemical practice
- •4.5.1 Phases and phase transitions of the lattice gas
- •4.5.4 Chemisorption and catalysis: macroeconomics, macromolecules and microscopy
- •Further reading for chapter 4
- •Problems and projects for chapter 4
- •5.1 Introduction: growth modes and nucleation barriers
- •5.1.1 Why are we studying epitaxial growth?
- •5.1.3 Growth modes and adsorption isotherms
- •5.1.4 Nucleation barriers in classical and atomistic models
- •5.2 Atomistic models and rate equations
- •5.2.1 Rate equations, controlling energies, and simulations
- •5.2.2 Elements of rate equation models
- •5.2.3 Regimes of condensation
- •5.2.4 General equations for the maximum cluster density
- •5.2.5 Comments on individual treatments
- •5.3 Metal nucleation and growth on insulating substrates
- •5.3.1 Microscopy of island growth: metals on alkali halides
- •5.3.2 Metals on insulators: checks and complications
- •5.4 Metal deposition studied by UHV microscopies
- •5.4.2 FIM studies of surface diffusion on metals
- •5.4.3 Energies from STM and other techniques
- •5.5 Steps, ripening and interdiffusion
- •5.5.2 Steps as sources: diffusion and Ostwald ripening
- •5.5.3 Interdiffusion in magnetic multilayers
- •Further reading for chapter 5
- •Problems and projects for chapter 5
- •6.1 The electron gas: work function, surface structure and energy
- •6.1.1 Free electron models and density functionals
- •6.1.2 Beyond free electrons: work function, surface structure and energy
- •6.1.3 Values of the work function
- •6.1.4 Values of the surface energy
- •6.2 Electron emission processes
- •6.2.1 Thermionic emission
- •6.2.4 Secondary electron emission
- •6.3.1 Symmetry, symmetry breaking and phase transitions
- •6.3.3 Magnetic surface techniques
- •6.3.4 Theories and applications of surface magnetism
- •Further reading for chapter 6
- •Problems and projects for chapter 6
- •7.1.1 Bonding in diamond, graphite, Si, Ge, GaAs, etc.
- •7.1.2 Simple concepts versus detailed computations
- •7.2 Case studies of reconstructed semiconductor surfaces
- •7.2.2 GaAs(111), a polar surface
- •7.2.3 Si and Ge(111): why are they so different?
- •7.2.4 Si, Ge and GaAs(001), steps and growth
- •7.3.1 Thermodynamic and elasticity studies of surfaces
- •7.3.2 Growth on Si(001)
- •7.3.3 Strained layer epitaxy: Ge/Si(001) and Si/Ge(001)
- •7.3.4 Growth of compound semiconductors
- •Further reading for chapter 7
- •Problems and projects for chapter 7
- •8.1 Metals and oxides in contact with semiconductors
- •8.1.1 Band bending and rectifying contacts at semiconductor surfaces
- •8.1.2 Simple models of the depletion region
- •8.1.3 Techniques for analyzing semiconductor interfaces
- •8.2 Semiconductor heterojunctions and devices
- •8.2.1 Origins of Schottky barrier heights
- •8.2.2 Semiconductor heterostructures and band offsets
- •8.3.1 Conductivity, resistivity and the relaxation time
- •8.3.2 Scattering at surfaces and interfaces in nanostructures
- •8.3.3 Spin dependent scattering and magnetic multilayer devices
- •8.4 Chemical routes to manufacturing
- •8.4.4 Combinatorial materials development and analysis
- •Further reading for chapter 8
- •9.1 Electromigration and other degradation effects in nanostructures
- •9.2 What do the various disciplines bring to the table?
- •9.3 What has been left out: future sources of information
- •References
- •Index
2.5 Thin ®lm deposition procedures |
57 |
|
|
lasers. Laser ablation, sometimes called pulsed laser deposition (PLD), has typically been used to deposit ceramic materials, including high temperature superconductors (Dijkamp et al. 1987, Venkatasan et al. 1988, Morimoto & Shimizu 1995); it produces very rapid deposition in which whole chunks of material can break oV and be deposited during the immense peak powers which typically last for 10±20 ns. A particular advantage of rapid evaporation is the control of stoichiometry, since the diVerent species do not have time to segregate to the surface during the evaporation phase.
2.5.3Molecular beam epitaxy and related methods
The large scale use of such evaporation sources, especially for depositing semiconductor or metallic multilayers, has become known as molecular beam epitaxy (MBE). This acroymn has spawned several sub-cases, such as GSMBE (Gas Source MBE), and MOMBE (Metal±Organic MBE) which is sometimes called CBE (chemical beam epitaxy). Thus MBE really spans a range of techniques which range from being merely a fancy name for thermal evaporation, to much more chemically oriented ¯ow process techniques such as chemical vapor deposition (CVD) and MOCVD which we describe brie¯y in section 2.5.5. MBE is covered in several books (e.g. Parker 1985, Tsao 1993, Glocker & Shah 1995) and in many review articles and ongoing conference series. Only a few general points can usefully be made here, but this preparation method lies behind much of the science discussed later in chapters 5±8.
The growth end of such a chamber used for GSMBE or MOMBE is shown schematically in ®gure 2.8 (Abernathy 1995). The halfspace in front of the substrate is a bank of eVusion cells and various forms of gas injector cells, which transport reacting chemicals to the substrate. The growth of III±V compounds such as GaAs or AlGaAs has been pursued using such techniques, starting from metal±alkyl compounds such as
triethylaluminum (TEA) and triethylgallium (TEG) and hydrides such as AsH3. The group ®ve hydrides are injected via cracker cells which convert them catalytically into
As2 and hydrogen, which then impinge on the substrate to react with the alkyl beam to produce the growing ®lm (Panish & Sumski 1984, Abernathy 1995).
These cells are surrounded by liquid nitrogen cooled shrouds, which both condense unwanted evaporants and improve the vacuum in the sample region, which is monitored by the mass spectrometer. One of the advantages of MBE and related methods is the relative ease by which in situ diagnostic tools can be incorporated into the vacuum system. The most widely used are RHEED, shown in ®gure 2.8 and described in section 3.2.2, plus various optical techniques, some of which can also work in higher pressure environments. A compendium of these `real-time diagnostics', particularly in the context of semiconductor growth, is given by several authors in Glocker & Shah (1995, part D).
2.5.4Sputtering and ion beam assisted deposition
There are many uses for ions in connection with the production of thin ®lms. Sputtering using relatively low energy (100 eV±2 keV) ions is often used for cleaning samples, while higher energy (5±200 keV) ions can be used for doping layers with
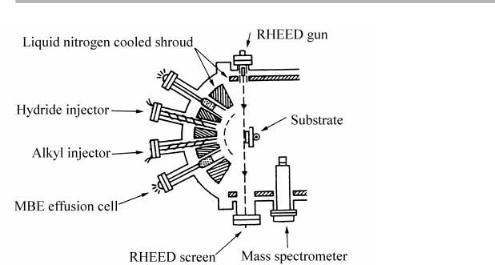
58 2 Surfaces in vacuum
Figure 2.8. GSMBE or MOMBE growth chamber containing eVusion and cracker cells, and RHEED diagnostics (after Abernathy 1995, reproduced with permission). See text for discussion.
electrically active impurities. Ions can also be used for deposition, where individual ions, or charged clusters can be deposited at a range of energies. Clearly, the fact that the ions are charged allows extra control, and there are various methods by which this can be done. Directed ion beams from an accelerator form the most obvious possibility, but plasma and magnetron sources are also widely used (Bunshah 1991, Vossen & Kern 1991, Shah 1995, Smith 1995).
One of the main claims for IBAD (Ion Beam Assisted Deposition) or IBSD (Ion Beam Sputter Deposition) is that better quality deposits can be obtained at lower substrate temperatures, thus avoiding large scale interdiVusion which results from high temperature processing. However, the deposited ®lms adhere well to the substrate because of the localized limited mixing caused by the ion impacts (Itoh 1989, 1995, Marton 1994). This is one example of device engineers trying to reduce the `thermal budget' and so produce sharper interfaces between dissimilar components. Another possibilty is to produce clusters in a supersonic expansion source, and to ionize them, controlling their ¯ight during cluster depostion with applied voltages. This has been termed ICB (Ionized Cluster Beam) technology by the inventers (Takagi 1986, Takagi & Yamada 1989). Yet another possibility is to use ion beams to react with the substrate and grow compounds such as oxides or nitrides at and near surfaces (Herbots et al. 1994).
All of these procedures are inherently complex; for almost all purposes the question is whether they produce `better' ®lms for particular applications, i.e. whether they give a large enough improvement over existing methods to justify the considerable investment involved. Although our aim here is to give an outline description, we will not pursue models of how of these methods work in any detail; they are all rather speci®c to the combination of material and ion beam technique, and whether the processes are reactive, in the sense of involving (ion) chemistry, or physical, meaning that only collisions and clustering are involved. Nonetheless, ion beam assisted methods are very

2.5 Thin ®lm deposition procedures |
59 |
|
|
|
reactor wall |
|
|
input |
|
output |
|
H2 |
gas phase reactions |
|
|
SiH4 |
diffusion |
surface |
diffusion |
|
|
|
|
|
adsorption |
processes |
desorption |
(a)
Growth rate
(b)
substrate
surface gas kinetics
transport
thermodynamics
gas velocity
Figure 2.9. (a) Schematic diagram of reactions involved in the CVD of silicon from silane (SiH4) and hydrogen (H2); (b) typical variation of the growth rate on gas velocity with rate limiting steps indicated (after Vescan 1995, reproduced with permission).
widespread and are of great economic importance; sputter deposition in particular has high throughput for the production of (textured) polycrystalline thin ®lms of a wide variety of materials (Bunshah 1991, Vossen & Kern 1991, Shah 1995). The language used to describe such ion based processes (see Greene 1991) necessarily starts from a similar vantage point to that used to describe thermal evaporation; the latter topic is treated here in detail in chapter 5.
2.5.5Chemical vapor deposition techniques
The growth of semiconductor layers for device production is most frequently done by a variant of CVD (Chemical Vapor Deposition). This is usually implemented as a ¯ow technique in which the reacting gases pass over a heated substrate, as indicated in ®gure 2.9(a) for growth of silicon from silane and hydrogen. CVD reactors are either of the hot wall or cold wall type, and are surrounded by an extended gas handling and pumping system with rigorous control of the (often highly toxic) gases (Carlsson 1991, Vescan 1995). Because the pressure may be up to an atmosphere in certain cases (APCVD: Atmospheric Pressure CVD) the growth rate can be high, and UHV technology is not an absolute necessity; but control of impurities is a dominant problem, as pointed out in section 2.4.4 (O'Hanlon 1994). Thus UHV-CVD is becoming more widespread, where
the total pressure is ,1 mbar. However, most commercial processing corresponds to