
- •Biological and Medical Physics, Biomedical Engineering
- •Medical Image Processing
- •Preface
- •Contents
- •Contributors
- •1.1 Medical Image Processing
- •1.2 Techniques
- •1.3 Applications
- •1.4 The Contribution of This Book
- •References
- •2.1 Introduction
- •2.2 MATLAB and DIPimage
- •2.2.1 The Basics
- •2.2.2 Interactive Examination of an Image
- •2.2.3 Filtering and Measuring
- •2.2.4 Scripting
- •2.3 Cervical Cancer and the Pap Smear
- •2.4 An Interactive, Partial History of Automated Cervical Cytology
- •2.5 The Future of Automated Cytology
- •2.6 Conclusions
- •References
- •3.1 The Need for Seed-Driven Segmentation
- •3.1.1 Image Analysis and Computer Vision
- •3.1.2 Objects Are Semantically Consistent
- •3.1.3 A Separation of Powers
- •3.1.4 Desirable Properties of Seeded Segmentation Methods
- •3.2 A Review of Segmentation Techniques
- •3.2.1 Pixel Selection
- •3.2.2 Contour Tracking
- •3.2.3 Statistical Methods
- •3.2.4 Continuous Optimization Methods
- •3.2.4.1 Active Contours
- •3.2.4.2 Level Sets
- •3.2.4.3 Geodesic Active Contours
- •3.2.5 Graph-Based Methods
- •3.2.5.1 Graph Cuts
- •3.2.5.2 Random Walkers
- •3.2.5.3 Watershed
- •3.2.6 Generic Models for Segmentation
- •3.2.6.1 Continuous Models
- •3.2.6.2 Hierarchical Models
- •3.2.6.3 Combinations
- •3.3 A Unifying Framework for Discrete Seeded Segmentation
- •3.3.1 Discrete Optimization
- •3.3.2 A Unifying Framework
- •3.3.3 Power Watershed
- •3.4 Globally Optimum Continuous Segmentation Methods
- •3.4.1 Dealing with Noise and Artifacts
- •3.4.2 Globally Optimal Geodesic Active Contour
- •3.4.3 Maximal Continuous Flows and Total Variation
- •3.5 Comparison and Discussion
- •3.6 Conclusion and Future Work
- •References
- •4.1 Introduction
- •4.2 Deformable Models
- •4.2.1 Point-Based Snake
- •4.2.1.1 User Constraint Energy
- •4.2.1.2 Snake Optimization Method
- •4.2.2 Parametric Deformable Models
- •4.2.3 Geometric Deformable Models (Active Contours)
- •4.2.3.1 Curve Evolution
- •4.2.3.2 Level Set Concept
- •4.2.3.3 Geodesic Active Contour
- •4.2.3.4 Chan–Vese Deformable Model
- •4.3 Comparison of Deformable Models
- •4.4 Applications
- •4.4.1 Bone Surface Extraction from Ultrasound
- •4.4.2 Spinal Cord Segmentation
- •4.4.2.1 Spinal Cord Measurements
- •4.4.2.2 Segmentation Using Geodesic Active Contour
- •4.5 Conclusion
- •References
- •5.1 Introduction
- •5.2 Imaging Body Fat
- •5.3 Image Artifacts and Their Impact on Segmentation
- •5.3.1 Partial Volume Effect
- •5.3.2 Intensity Inhomogeneities
- •5.4 Overview of Segmentation Techniques Used to Isolate Fat
- •5.4.1 Thresholding
- •5.4.2 Selecting the Optimum Threshold
- •5.4.3 Gaussian Mixture Model
- •5.4.4 Region Growing
- •5.4.5 Adaptive Thresholding
- •5.4.6 Segmentation Using Overlapping Mosaics
- •5.6 Conclusions
- •References
- •6.1 Introduction
- •6.2 Clinical Context
- •6.3 Vessel Segmentation
- •6.3.1 Survey of Vessel Segmentation Methods
- •6.3.1.1 General Overview
- •6.3.1.2 Region-Growing Methods
- •6.3.1.3 Differential Analysis
- •6.3.1.4 Model-Based Filtering
- •6.3.1.5 Deformable Models
- •6.3.1.6 Statistical Approaches
- •6.3.1.7 Path Finding
- •6.3.1.8 Tracking Methods
- •6.3.1.9 Mathematical Morphology Methods
- •6.3.1.10 Hybrid Methods
- •6.4 Vessel Modeling
- •6.4.1 Motivation
- •6.4.1.1 Context
- •6.4.1.2 Usefulness
- •6.4.2 Deterministic Atlases
- •6.4.2.1 Pioneering Works
- •6.4.2.2 Graph-Based and Geometric Atlases
- •6.4.3 Statistical Atlases
- •6.4.3.1 Anatomical Variability Handling
- •6.4.3.2 Recent Works
- •References
- •7.1 Introduction
- •7.2 Linear Structure Detection Methods
- •7.3.1 CCM for Imaging Diabetic Peripheral Neuropathy
- •7.3.2 CCM Image Characteristics and Noise Artifacts
- •7.4.1 Foreground and Background Adaptive Models
- •7.4.2 Local Orientation and Parameter Estimation
- •7.4.3 Separation of Nerve Fiber and Background Responses
- •7.4.4 Postprocessing the Enhanced-Contrast Image
- •7.5 Quantitative Analysis and Evaluation of Linear Structure Detection Methods
- •7.5.1 Methodology of Evaluation
- •7.5.2 Database and Experiment Setup
- •7.5.3 Nerve Fiber Detection Comparison Results
- •7.5.4 Evaluation of Clinical Utility
- •7.6 Conclusion
- •References
- •8.1 Introduction
- •8.2 Methods
- •8.2.1 Linear Feature Detection by MDNMS
- •8.2.2 Check Intensities Within 1D Window
- •8.2.3 Finding Features Next to Each Other
- •8.2.4 Gap Linking for Linear Features
- •8.2.5 Quantifying Branching Structures
- •8.3 Linear Feature Detection on GPUs
- •8.3.1 Overview of GPUs and Execution Models
- •8.3.2 Linear Feature Detection Performance Analysis
- •8.3.3 Parallel MDNMS on GPUs
- •8.3.5 Results for GPU Linear Feature Detection
- •8.4.1 Architecture and Implementation
- •8.4.2 HCA-Vision Features
- •8.4.3 Linear Feature Detection and Analysis Results
- •8.5 Selected Applications
- •8.5.1 Neurite Tracing for Drug Discovery and Functional Genomics
- •8.5.2 Using Linear Features to Quantify Astrocyte Morphology
- •8.5.3 Separating Adjacent Bacteria Under Phase Contrast Microscopy
- •8.6 Perspectives and Conclusions
- •References
- •9.1 Introduction
- •9.2 Bone Imaging Modalities
- •9.2.1 X-Ray Projection Imaging
- •9.2.2 Computed Tomography
- •9.2.3 Magnetic Resonance Imaging
- •9.2.4 Ultrasound Imaging
- •9.3 Quantifying the Microarchitecture of Trabecular Bone
- •9.3.1 Bone Morphometric Quantities
- •9.3.2 Texture Analysis
- •9.3.3 Frequency-Domain Methods
- •9.3.4 Use of Fractal Dimension Estimators for Texture Analysis
- •9.3.4.1 Frequency-Domain Estimation of the Fractal Dimension
- •9.3.4.2 Lacunarity
- •9.3.4.3 Lacunarity Parameters
- •9.3.5 Computer Modeling of Biomechanical Properties
- •9.4 Trends in Imaging of Bone
- •References
- •10.1 Introduction
- •10.1.1 Adolescent Idiopathic Scoliosis
- •10.2 Imaging Modalities Used for Spinal Deformity Assessment
- •10.2.1 Current Clinical Practice: The Cobb Angle
- •10.2.2 An Alternative: The Ferguson Angle
- •10.3 Image Processing Methods
- •10.3.1 Previous Studies
- •10.3.2 Discrete and Continuum Functions for Spinal Curvature
- •10.3.3 Tortuosity
- •10.4 Assessment of Image Processing Methods
- •10.4.1 Patient Dataset and Image Processing
- •10.4.2 Results and Discussion
- •10.5 Summary
- •References
- •11.1 Introduction
- •11.2 Retinal Imaging
- •11.2.1 Features of a Retinal Image
- •11.2.2 The Reason for Automated Retinal Analysis
- •11.2.3 Acquisition of Retinal Images
- •11.3 Preprocessing of Retinal Images
- •11.4 Lesion Based Detection
- •11.4.1 Matched Filtering for Blood Vessel Segmentation
- •11.4.2 Morphological Operators in Retinal Imaging
- •11.5 Global Analysis of Retinal Vessel Patterns
- •11.6 Conclusion
- •References
- •12.1 Introduction
- •12.1.1 The Progression of Diabetic Retinopathy
- •12.2 Automated Detection of Diabetic Retinopathy
- •12.2.1 Automated Detection of Microaneurysms
- •12.3 Image Databases
- •12.4 Tortuosity
- •12.4.1 Tortuosity Metrics
- •12.5 Tracing Retinal Vessels
- •12.5.1 NeuronJ
- •12.5.2 Other Software Packages
- •12.6 Experimental Results and Discussion
- •12.7 Summary and Future Work
- •References
- •13.1 Introduction
- •13.2 Volumetric Image Visualization Methods
- •13.2.1 Multiplanar Reformation (2D slicing)
- •13.2.2 Surface-Based Rendering
- •13.2.3 Volumetric Rendering
- •13.3 Volume Rendering Principles
- •13.3.1 Optical Models
- •13.3.2 Color and Opacity Mapping
- •13.3.2.2 Transfer Function
- •13.3.3 Composition
- •13.3.4 Volume Illumination and Illustration
- •13.4 Software-Based Raycasting
- •13.4.1 Applications and Improvements
- •13.5 Splatting Algorithms
- •13.5.1 Performance Analysis
- •13.5.2 Applications and Improvements
- •13.6 Shell Rendering
- •13.6.1 Application and Improvements
- •13.7 Texture Mapping
- •13.7.1 Performance Analysis
- •13.7.2 Applications
- •13.7.3 Improvements
- •13.7.3.1 Shading Inclusion
- •13.7.3.2 Empty Space Skipping
- •13.8 Discussion and Outlook
- •References
- •14.1 Introduction
- •14.1.1 Magnetic Resonance Imaging
- •14.1.2 Compressed Sensing
- •14.1.3 The Role of Prior Knowledge
- •14.2 Sparsity in MRI Images
- •14.2.1 Characteristics of MR Images (Prior Knowledge)
- •14.2.2 Choice of Transform
- •14.2.3 Use of Data Ordering
- •14.3 Theory of Compressed Sensing
- •14.3.1 Data Acquisition
- •14.3.2 Signal Recovery
- •14.4 Progress in Sparse Sampling for MRI
- •14.4.1 Review of Results from the Literature
- •14.4.2 Results from Our Work
- •14.4.2.1 PECS
- •14.4.2.2 SENSECS
- •14.4.2.3 PECS Applied to CE-MRA
- •14.5 Prospects for Future Developments
- •References
- •15.1 Introduction
- •15.2 Acquisition of DT Images
- •15.2.1 Fundamentals of DTI
- •15.2.2 The Pulsed Field Gradient Spin Echo (PFGSE) Method
- •15.2.3 Diffusion Imaging Sequences
- •15.2.4 Example: Anisotropic Diffusion of Water in the Eye Lens
- •15.2.5 Data Acquisition
- •15.3 Digital Processing of DT Images
- •15.3.2 Diagonalization of the DT
- •15.3.3 Gradient Calibration Factors
- •15.3.4 Sorting Bias
- •15.3.5 Fractional Anisotropy
- •15.3.6 Other Anisotropy Metrics
- •15.4 Applications of DTI to Articular Cartilage
- •15.4.1 Bovine AC
- •15.4.2 Human AC
- •References
- •Index

240 |
C. Adam and G. Dougherty |
10.4 Assessment of Image Processing Methods
Before adopting an automated method for the assessment of spinal deformities, there is a need to compare the proposed method with the results from current practice, in particular the Cobb angle which is the clinically accepted measure of scoliosis severity. Here, we apply the image processing techniques described above to derive Cobb-equivalent and tortuosity metrics in a series of AIS patients. We then compare the new metrics with existing, clinically measured Cobb angles for the patients in the series.
10.4.1 Patient Dataset and Image Processing
The patient group comprised 79 AIS patients from the Mater Children’s Hospital in Brisbane, Australia. Each of the patients in this series underwent thoracoscopic (keyhole) anterior surgery for correction of their deformity, and prior to surgery each patient received a single, low-dose, pre-operative CT scan for surgical planning purposes. Pre-operative CT allows safer screw sizing and positioning in keyhole scoliosis surgery procedures [56]. The estimated CT radiation dose for the scanning protocol used was 3.7 mSv [57].
Following the procedure described in Sect. 10.3.2, vertebral canal landmark coordinates were measured for each thoracolumbar vertebrae in each patient in the series. We note that measurement of spinal deformities from supine CT scans yields lower values of the Cobb angle than measurements on standing patients. Torell et al. [58] reported a 9◦ reduction in Cobb angle for supine compared to standing patients, and Yazici et al. [59] showed a reduction in average Cobb angle from 56 to 39◦ between standing and supine positions.
10.4.2 Results and Discussion
The patient group comprised of 74 females and 5 males with a mean age of 15.6 years (range 9.9–41.2) at the time the CT scan was performed. The mean height was 161.5 cm (range 139.5–175) and mean weight was 53.4 kg (range 30.6–84.7). All 79 patients had right-sided major scoliotic curves.1 The mean clinically measured major Cobb angle for the group was 51.9◦ (range 38–68◦). The clinical Cobb measurements were performed manually at the Mater Children’s Hospital spinal clinic by experienced clinicians, using standing radiographs. Figure 10.12 shows a
1The major curve is defined as the curve with the largest Cobb angle in a scoliotic spine. Typically, adolescent idiopathic scoliosis major curves are convex to the right in the mid-thoracic spine, with smaller (minor) curves above and below, convex to the left.
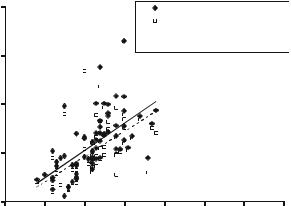
10 Applications of Medical Image Processing in the Diagnosis and Treatment... |
241 |
Cobb-equivalent angle (°)
100 |
Cobb-equivalent 1 |
Cobb-equivalent 2
Linear (Cobb-equivalent 1)
Linear (Cobb-equivalent 2)
80
y = 1.13 x -15.52 R² = 0.38
60
y = 1.04 x -13.72 R² = 0.32
40
20 |
|
|
|
|
|
|
|
30 |
40 |
50 |
60 |
70 |
80 |
90 |
100 |
Clinically measured Cobb (°)
Fig. 10.12 Plot of Cobb-equivalent 1 and Cobb-equivalent 2 angles against clinically measured coronal Cobb angle for each patient in the series. Note that for 10 of the 79 patients, there was only one inflection point on the polynomial curve so Cobb-equivalent1 could not be determined for these ten patients
comparison between the clinically measured (standing) Cobb angle and the Cobbequivalent 1 and Cobb-equivalent 2 metrics derived from the supine CT scans for the patient group.
With respect to Fig. 10.12, the relatively low R2 values of 0.38 and 0.32 (Cobb-equivalent 1 and Cobb-equivalent 2, respectively) show there are substantial variations between individual clinical Cobb measurements from standing radiographs, and the Cobb-equivalent angles derived from continuum representations of spine shape on supine CT scans. The 13.7 and 15.5◦ offsets in the linear regression equations for the two Cobb-equivalent angles are consistent with the magnitude of change in Cobb angle between standing and supine postures of 9◦ and 17◦ reported by Torell et al. [58] and Yazici et al. [59]. The gradients of the regression lines for Cobb-equivalent 1 (1.13) and Cobb-equivalent 2 (1.04) in Fig. 10.13 are close to unity as would be expected, but the slightly greater than unity values suggest that either (1) bigger scoliotic curves are more affected by gravity (i.e. the difference between standing and supine Cobb increases with increasing Cobb angle), or (2) there is greater rotation of the anterior vertebral column (where clinical Cobb angles are measured from endplates) compared to the posterior vertebral canal (where the Cobb-equivalent landmarks are measured) in patients with larger deformities. Note that although not shown in Fig. 10.12, Cobb-equivalent 1 and Cobb-equivalent 2 are highly correlated with each other (R2 = 0.96).
Figure 10.13 shows the close correlation between two of the new metrics, the coronal tortuosity (segmental Ferguson angle) of the major curve and the Cobb-equivalent 2. The coronal tortuosity, M (or segmental Ferguson angle), is strongly correlated (R2 = 0.906, p < 0.0000001) with the Cobb-equivalent 2 angle.
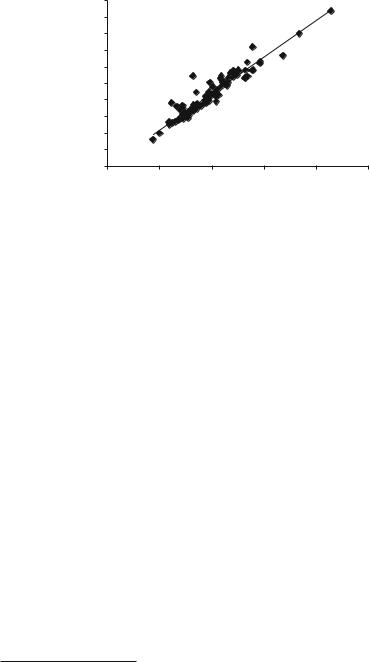
242
Major Coronal Tortuosity (°)
C. Adam and G. Dougherty
100.0 |
|
|
|
|
|
90.0 |
|
|
|
|
|
80.0 |
|
|
|
|
|
70.0 |
|
|
|
y = 1.0985x |
|
60.0 |
|
|
|
|
|
|
|
|
R² = 0.9057 |
|
|
50.0 |
|
|
|
|
|
40.0 |
|
|
|
|
|
30.0 |
|
|
|
|
|
20.0 |
|
|
|
|
|
10.0 |
|
|
|
|
|
0.0 |
|
|
|
|
|
0.0 |
20.0 |
40.0 |
60.0 |
80.0 |
100.0 |
Cobb-Equivalent 2 (°)
Fig. 10.13 Major coronal tortuosity (segmental Ferguson angle) vs. Cobb-equivalent 2 for the patient group, showing the close correlation between these two metrics
It is almost 10% larger for all angles, as expected from a metric based on the Ferguson angle, and there is no offset angle. This represents a strong internal consistency for these two semi-automated metrics. M follows the Cobb-equivalent 2 angle in being larger than the measured Cobb angle and having a significant correlation (R2 = 0.30, p < 0.007) to it.
To remove the influence of (1) patient positioning (supine vs. standing) and
(2) measurement error associated with a single clinical Cobb measurement, we performed a separate sub-study on 12 of the 79 patients in the main patient group.2 For each patient in the sub-study, repeated manual Cobb measurements (six clinicians measured each Cobb angle on three separate occasions at least a week apart) were made using 2D coronal reconstructions from the supine CT scans. This allowed direct comparison of manual and Cobb-equivalent metrics using the same supine CT datasets, and reduced manual measurement variability by using repeated measures by multiple observers. Figure 10.14 shows the result of this comparison.
The R2 value of 0.88 in Fig. 10.14 suggests that when postural differences are accounted for, the Cobb-equivalent metric is strongly correlated to manual Cobb measurements, but has the advantage of not being prone to the substantial manual measurement variability which can occur with a single Cobb measurement by a single observer. Note that the intercept and gradient of the regression equation in Fig. 10.14 suggest that although the metric is strongly correlated with supine manual measures, there is still a difference between the magnitudes of the two Cobb measures, perhaps due to the difference in anatomical location of the landmarks used in each case (manual Cobb uses endplates in the anterior column, whereas
2Note that the measurements described in this sub-study were performed before the main study, so there was no bias in the selection of the 12 patients based on the results from the entire patient group.
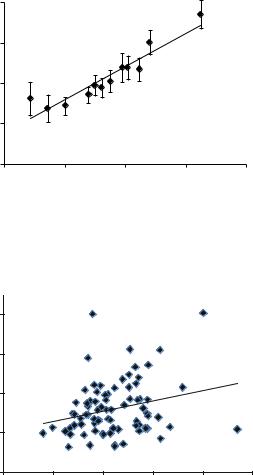
10 Applications of Medical Image Processing in the Diagnosis and Treatment... |
243 |
|||||
CT |
60 |
|
|
|
|
|
|
|
|
|
|
|
|
supinefromCobbManual reconstructions(°) |
50 |
|
|
|
|
|
|
|
|
|
|
|
|
|
|
|
|
y = 0.8192x + 11.37 |
|
|
|
|
|
|
R² = 0.8812 |
|
|
|
40 |
|
|
|
|
|
|
30 |
|
|
|
|
|
|
20 |
|
|
|
|
|
|
20 |
30 |
40 |
50 |
60 |
|
|
|
|
Cobb-equivalent 1 (°) |
|
|
Fig. 10.14 Comparison of manually measured Cobb and Cobb-equivalent 1 for a subgroup of 12 patients, where manual Cobb angles were measured from 2D coronal supine CT reconstructions. Each data point represents the mean of 18 manual measurements (six observers on three occasions each). Error bars are the standard deviation of the manual measurements
tortuosity, |
800 |
|
|
|
|
|
|
|
|
|
|
|
|
rms |
600 |
|
|
|
y = 2.6057x + 208.65 |
|
|
|
|
|
R² = 0.0642 |
||
normalized |
|
|
|
|
||
400 |
|
|
|
|
|
|
K |
|
|
|
|
|
|
Coronal |
200 |
|
|
|
|
|
0 |
|
|
|
|
|
|
|
0.0 |
20.0 |
40.0 |
60.0 |
80.0 |
100.0 |
Coronal tortuosity, M
Fig. 10.15 Plot of normalized root-mean-square tortuosity, K, against tortuosity, M, both measured in the coronal (AP) plane, for each patient in the series
Cobb-equivalent metric uses vertebral canal). Also the number of patients used in this sub-study was relatively small, due to the constraints associated with obtaining multiple repeat Cobb measurements by a group of clinicians.
Figure 10.15 shows the relationship between the two tortuosity-based metrics, K and M (Sect. 10.3.3). Clearly, the correlation is very poor, although both metrics have been shown to correlate well with the ranking of an expert panel when used with retinal vessels [47]. However, in this application, the data is very sparse and there are no ‘data balls’ which can be used to constrain the spline-fitting [45].