
Multidimensional Chromatography
.pdf
PREFACE
Separation Science is a mature and unified subject in which now conventional chromatographic and electrically driven processes are applied in the analysis of mixtures of compounds ranging from permanent gases to proteins. The boundaries between previously distinct techniques are increasingly blurred and it is becoming very evident that is a single theory may be applicable to chromatography whatever the physical state of the mobile phase. Gas, liquid and supercritical fluid chromatography can be regarded as special cases of the same procedure, while capillary electrochromatography combines liquid chromatography with electrophoresis.
Separation science is now very focused on reducing not only timescales for analyzis, but also the size and physical nature of the analytical device, Miniaturisation of entire analytical procedures provides a strong driving force for these trends in unifying theory and practice, and is a process likely to continue, as separations using microfluidic devices are developed. In spite of these many advances however, the complexity of many naturally occurring mixtures exceeds the capacity of any single method, even when optimized to resolve them. For many years therefore, intense effort has been concentrated on coupling separations methods together to increase resolution, and these have proceeded parallel with advances in coupling separation methods with spectroscopy. As our ability to isolate components in mixtures has increased, so has our appreciation for the shear complexity of compounds found in nature, Even separation systems with the capacity to isolate many thousands of species, are found to be inadequate when applied to commonplace mixtures such as diesel fuel. We clearly have some way to go in realising separation systems that can provide truly universal and complete separations.
Recent advances in multidimensional separation methods have been rapid and we considered that the time was appropriate to bring together accounts by leading researchers who are developing and applying multidimensional techniques. These authors have emphasized underlying theory along with instrumentation and practicalities, and have illustrated techniques with real-world examples. We hope that the eader will be as excited as we are by this combined account of progress. We thank all our contributors for their significant efforts in producing chapters of high scientific quality. We are especially indebted to Katya Vines of John Wiley who guided the project through its early stages and more recently to Emma Dowdle who brought it to completion.
KEITH BARTLE, Leeds ALLY LEWIS, Leeds LUIGI MONDELLO, Messina

Multidimensional Chromatography
Edited by Luigi Mondello, Alastair C. Lewis and Keith D. Bartle
Copyright © 2002 John Wiley & Sons Ltd
ISBNs: 0-471-98869-3 (Hardback); 0-470-84577-5 (Electronic)
Index
A
Additives in polymers, analysis for 305–311, 324
Aniline, determination of impurities in 321 Aromatic hydrocarbons, determination of
343, 389, 394, 400
B
Bentazone, determination of 346 Biomedical applications 251–289 see also forensic applications
Breakthrough volume 121, 123
C
Capillary electrodriven separation techniques (CESTs) 143–146 Capillary electrophoresis 201–203,
285–290
Capillary gas chromatography, hot injectors 25–27
Capillary zone electrophoresis 201–212 Chiral forms, determination of 69–71, 126,
218–222
Co-solvent trapping 25, 29 Cold trapping 20
Combustion product analysis 59, 61 – 62 Comprehensive gas chromatography 79–82,
89–92, 397–401
Measurement of performance 102–103 see also gas chromatography
Comprehensive (coupled) liquid chromatography 253–254
Concurrent eluent evaporation 22–25, 26, 28–29
see also retention gap method Concurrent solvent evaporation see
concurrent eluent evaporation Coupled-column liquid chromatography recycling chromatographic system
127–129
separation system 123–129
theory of 111–116
Cryogenic modulator 84–94, 103
D
Deans switching 52–55 see also heart-cuts
Diesel fuel, determination of aromatic hydrocarbons 394, 395
Dimethyl sulfide 339–349, 342 Drug analysis 410–411
E
Electrophoresis
capillary techniques with HPLC 201–203, 204–212
two-dimensional comprehensive separations 200–212
see also capillary electrodriven separation techniques (CESTs)
Enantiomers, separation of see chiral forms End-cuts see heart-cuts
Enhanced fluidity liquid chromatography (EFLC) 156, 157
Environmental analysis 62, 68–71, 335–370 examples 347–348
Ethylene-thiourea (ETU), determination 346
F
Fire debris 418
Flavor analysis see food analysis Food analysis 62–68
HPLC 231–234
HPLC-HRGC coupling 235–243 MDGC 217 – 231, 422
TLC 242–245
Food packaging 305–306 Forensic applications 407–429
see also biomedical applications Fragrance analysis see food analysis
434 |
Index |
Front-cuts see heart-cuts
Fungicide determination 340, 346, 411–412
G
Gas chromatography applications 317–322 solvating GC 158
see also comprehensive gas chromatography; high performance liquid chromatography (HPLC), coupling with HRGC; multidimensional gas chromatography (MDGC); supercritical fluid chromatography (SFC); supercritical fluid techniques; two-dimensional gas chromatography
Gasoline analysis 325–328, 389–392 General elution problem 112–113 Gradient elution 244
Grafted planar chromatography 186 – 188 see also overpressured layer chromatog-
raphy (OPLC); thin-layer chromatography (TLC), multidimensional planar chromatography (MD-PC)
Growth hormones 408, 410
H
Heart-cuts 11–12, 78–79, 123–125, 254–265
see also Deans switching; multidimensional liquid chromatography (MDLC)
Herbicides see pesticides Heteromodal LC-LC 117
High performance liquid chromatography (HPLC) 304 – 306
coupling with HRGC
in food analysis 235–243 transfer techniques 17–38, 19fig in food analysis 231–234
see also liquid chromatography (LC); micellar HPLC; multidimensional liquid chromatography
High resolution gas chromatography (HRGC) see high performance liquid chromatography (HPLC), coupling with HRGC; multidimensional gas chromatography (MDGC)
Homomodal LC-LC 117
Hot injectors for GC 25–27 Hydrocarbon analysis 394–395, 402
I
Immunoaffinity chromatography, coupled with RPLC 126
Immunoaffinity sorbents in SPE-LC 270 – 272
Industrial applications 303–331
see also oils, analysis of; petroleum analysis
Industrial materials, analysis for impurities 321 – 323
Isoprene, determination of 339–340, 342
L
Lignites, analysis of pyrolysis products 313–315
Liquid chromatography (LC) 265–273, 273–278, 358–362
analysis of aromatics in diesel fuel 394 coupled with CE 289–290 environmental applications 343–358,
362–370
growth hormones 408, 410 hydrocarbon determination 394 – 395 LC-LC coupling see coupled-column
liquid chromatography organochlorine determination 408–409 recycling system 127–129
sulfur determination 395–397 trace enrichment 117–123
see also high performance liquid chromatography (HPLC); multidimensional liquid chromatography (MDLC); normal phase liquid chromatography (NPLC); reversed phase liquid chromatography (RPLC)
Liquid-liquid extraction (LLE) 35–37, 40 Longitudinally modulated cryogenic system
(LMCS) see cryogenic modulator Loop-type interfaces see concurrent eluent
evaporation
M
Mass spectrometry, isotope ratio (IRMS) 223, 226, 228, 422–424
MDGC see multidimensional gas
Index |
435 |
chromatography (MDGC) Micellar HPLC 411
see also high performance liquid chromatography (HPLC)
Modulation technology 82–94 Multichromatography 77 Multidimensional chromatography see
entries beginning “multidimensional”; unified chromatography
Multidimensional gas chromatography (MDGC) 77 – 80
determination of aromatic hydrocarbons 388
determination of isoprene and dimethyl sulphide 339–340, 342
food and flavor analysis 217–231, 218–231 forensic and toxicological applications
414–427
heteromodal and homomodal 117 industrial applications 317–323 multiple traps 80
natural gas analysis 385–387 selected 87–89
sulfur determination in ethene and propene 381–383, 384
targeted 87–89
total olefin determination in gasolines 391, 393
wines and beverages 229–230
see also comprehensive gas chromatography; gas chromatography; high performance liquid chromatography (HPLC), coupling with HRGC
Multidimensional liquid chromatography (MDLC) 110def, 341–358, 410–414
in drug analysis 410 –411
in environmental analysis 341, 343–358 offand on-line modes 111
targeted mode in food analysis 254–265 see also comprehensive liquid chromatog-
raphy; liquid chromatography Multidimensional planar chromatography
(MD-PC) 172–173 combining methods 191–193
in food and flavor analysis 242–245 one-dimensional separation 178–182 selection of mobile phases 175–176 three-dimensional separation 184–185 two-dimensional separation 173–177,
182–184
see also grafted planar chromatography; overpressured layer chromatography
(OPLC); thin-layer chromatography (TLC)
N
Naphtha analysis see gasoline analysis Natural gas analysis by GC 385– 387 Normal phase liquid chromatography
(NPLC) 324
Normal phase liquid chromatography (NPLC) 273–276, 305–306, 324–325
O
Oils, analysis of 305–306, 425–426
see also industrial applications; petroleum analysis
Olefin determination in gasoline 391, 393 Open tubular trapping (OTT) 37–38, 41 OPLC see overpressured layer
chromatography (OPLC) Organochlorine compounds see entries
beginning “polychlorinated”; pesticides Organophosphorus compounds see pesticides Orthogonal gas chromatography
see orthogonality Orthogonality 94–101
tuning separations 96–97 Overpressured layer chromatography
(OPLC) 184–186, 188–191, 243–244 see also grafted planar chromatography; multidimensional planar chro-
matography (MD-PC); thin-layer chromatography (TLC)
P
Partially concurrent eluent evaporation 22, 25 Persistent organic pollutants (POPs) 68–71 Pesticides 238–242, 408–409, 426–427
see also environmental analysis Petroleum analysis 58–60
see also industrial applications; oils, analysis of
Pharmaceutical applications see biomedical applications
Phase-ratio focusing 18, 20 PIONA analysis 58, 389–391
Polychlorinated biphenyls (PCBs) 68–70, 240, 338–340, 343, 408–409, 417
Polychlorinated dibenzodioxins (PCDDs) 70, 343
436 |
Index |
Polychlorinated dibenzofurans (PCDFs) 70, 343
Polymer additives, analysis for 305–311, 324 Polymer applications 304–331
Pressure tuning experiment 77–78 Programmed multiple development (PMD)
244–245
Programmed temperature vaporizing (PTV) 25–27, 138, 339–340
R
Recycling chromatographic system 127–129
see also heart-cuts Refinery gas analysis 383–385
Resolution 4, 6–9, 10, 111–112
Retention gap method 18, 20–22, 23fig, 28 see also concurrent eluent evaporation
Reversed phase liquid chromatography (RPLC) 343–344
coupled with electrophoresis 201–203, 204–212
coupled to GC 31, 33–34, 277–279 environmental applications 345–357 immunoaffinity chromatography 126 transfer of water-bearing solvents to GC
column 28–38
rubber stocks, analysis of 315–317
S
Selected multidimensional gas chromatography (MDGC) 87–89
Separations, two-dimensional 9–12 Size exclusion chromatography (SEC)
306–315, 315–317
Solid phase extraction (SPE) 31, 33–34, 139–140, 142fig, 265–273, 278, 280–284, 286–289
Solid phase microextraction (SPME) see solid phase extraction (SPE)
Solvating gas chromatography 158 Subcritical fluid chromatography (subFC)
156, 157
Sulfur compounds
in ethene and propene 381–384
in middle distillates 395–397, 399, 400 Supercritical fluid chromatography (SFC)
325–330
analysis of aromatics in diesel fuel 394 see also supercritical fluid techniques
Supercritical fluid extraction (SFE)
see supercritical fluid chromatography (SFC); supercritical fluid
techniques
Supercritical fluid techniques 135–147, 241–242, 284–285
see also supercritical fluid chromatography (SFC)
T
Targeted multidimensional gas chromatography (MDGC) 87–89
Thermal sweeper 82–84 Thin-layer chromatography (TLC)
in food analysis 242–245
see also grafted planar chromatography; multidimensional planar chromatography (MD-PC); overpressured layer chromatography (OPLC)
Toxaphenes 70, 71
Toxicological applications 407–429 Tuning separations 96–97 Two-dimensional gas chromatography
applications 57–71 interfacing of columns 49–52 multiple-oven systems 56–57 refocusing of analytes 55–56
U
Unified chromatography 4–6, 147, 151–167
advantages and challenges 162–164 column efficiency and plate heights
164–167 instrumentation 159–162 phase diagram view 153–159
Multidimensional Chromatography
Edited by Luigi Mondello, Alastair C. Lewis and Keith D. Bartle Copyright © 2002 John Wiley & Sons Ltd ISBNs: 0-471-98869-3 (Hardback); 0-470-84577-5 (Electronic)
Part 1
General

Multidimensional Chromatography
Edited by Luigi Mondello, Alastair C. Lewis and Keith D. Bartle
Copyright © 2002 John Wiley & Sons Ltd
ISBNs: 0-471-98869-3 (Hardback); 0-470-84577-5 (Electronic)
1 Introduction
K.D. BARTLE
University of Leeds, Leeds, UK
1.1PREAMBLE
The natural world is one of complex mixtures: petroleum may contain 105–106 components, while it has been estimated that there are at least 150 000 different proteins in the human body. The separation methods necessary to cope with complexity of this kind are based on chromatography and electrophoresis, and it could be said that separation has been the science of the 20th century (1, 2). Indeed, separation science spans the century almost exactly. In the early 1900s, organic and natural product chemistry was dominated by synthesis and by structure determination by degradation, chemical reactions and elemental analysis; distillation, liquid extraction, and especially crystallization were the separation methods available to organic chemists.
Indeed, great emphasis was placed on the presentation of compounds in crystalline form; for many years, early chromatographic procedures for the separation of natural substances were criticized because the products were not crystalline. None the less, the invention by Tswett (3) of chromatographic separation by continuous adsorption/desorption on open columns as applied to plant extracts was taken up by a number of natural product researchers in the 1930s, notably by Karrer (4) and by Swab and Jockers (5). An early example (6) of hyphenation was the use of fluorescence spectroscopy to identify benzo[a]pyrene separated from shale oil by adsorption chromatography on alumina.
The great leap forward for chromatography was the seminal work of Martin and Synge (7) who in 1941 replaced countercurrent liquid – liquid extraction by partition chromatography for the analysis of amino acids from wool. Martin also realized that the mobile phase could be a gas rather than a liquid, and with James first developed (8) gas chromatography (GC) in 1951, following the gas-phase adsorption – chromatographic separations of Phillips (9).
Early partition chromatography was carried out on packed columns, but in 1958 Golay, in a piece of brilliant inductive reasoning (10), showed how a tortuous path through a packed bed could be replaced by a much straighter path through a narrow open tube. Long, and hence highly efficient columns for GC, could thus be fabricated from metal or glass capillaries, and remarkable separations were soon

4 |
Multidimensional Chromatography |
demonstrated. None the less, practical difficulties associated with capillary column technology generally restricted open tubular GC to a minority of applications until the fused silica column revolution in 1979. Dandeneau and Zerenner realized (11) that manufacturing methods for fibre-optic cables could be applied to make robust and durable capillary tubes with inactive inner surfaces. Lee et al. then delineated (12) the chemistry underlying the coating of such capillaries with a variety of stationary phases, and the age of modern high-resolution GC was born. Small diameter fused-silica capillaries were also found by Jorgenson and Lukacs (13) to be suitable for electrodriven separations since the heat generated could be readily dissipated because of the high surface-area-to-volume ratio. The invention of capillary supercritical fluid chromatography (SFC) in 1981 by Lee, Novotny and, co-workers (14) also depended on the availability of fused-silica capillary columns.
Liquid chromatography, however, took a different course, largely because slow diffusion in liquids meant that separations in open tubes necessitated inner diameters which were too small to make this approach practical. On the other hand, greatly increased efficiencies could be achieved on columns packed with small silica particles with bonded organic groups, and the technology for such columns was made available following the pioneering work of Horvath et al. (15) and Kirkland (16), thus giving rise to high performance liquid chromatography (HPLC). Even so, the available theoretical plate numbers (N) are limited in HPLC at normally accessible pressures and a different separation principle is therefore made use of. Since the resolution, R, for the separation of two compounds with retention factors k1 and k2 is given by:
R √N
4
|
1 2 k2 |
|
1 |
|
k |
(1.1)
where , the selectivity, is k2/k1, it follows that increased resolution based on column efficiency can only be achieved by very large increases in column length, because of the square-root dependence of R on N. However, a small increase in has a major influence on R, and selectivity is therefore the principal means of achieving separation in HPLC through the tremendous variety of differently bonded stationary phase groups.
1.2 PACKED CAPILLARY COLUMN AND UNIFIED CHROMATOGRAPHY
Small-diameter packed columns offer (17) the substantial advantages of small volumetric flow rates (1–20 ( L min 1)), which have environmental advantages, as well as permitting the use of ‘exotic’ or expensive mobile phases. Peak volumes are reduced (see Table 1.1), driven by the necessity of analysing the very small (picomole) amounts of substance available, for example, in small volumes of body fluids, or in the products of single-bead combinatorial chemistry.
The increasing use of microcolumns has moved chromatography towards unification. Giddings was the first to point out (18) that there was no distinction between
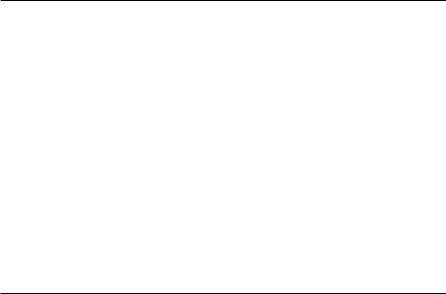
Introduction |
|
|
|
|
|
5 |
|
|
Table 1.1 Comparison of packed columns for analytical chromatography |
||||||
|
|
|
|
|
|
||
|
|
Volumetric |
Injection |
UV-detector |
Sensitivity |
||
Column internal diameter |
flow rate |
volume |
cell volume |
improvementa |
|||
4.6 mm (“conventional”) |
1 mL min 1 |
20 |
L |
8 L |
(1) |
||
1 mm (‘microbore’) |
50 |
L min 1 |
1 |
L |
400 nL |
21 |
|
250 |
m (“micro”) |
3 |
L min 1 |
60 nL |
25 nL |
340 |
|
75 |
m (‘nano’) |
300 nL min 1 |
5 nL |
3 nL |
3760 |
a Values are expressed relative to (conventional) 4.6 mm column.
chromatographic separation modes, which are classified according to the physical state of the mobile phase (GC, SFC or HPLC) but which move towards convergence as microcolumns are employed. Towards the end of the 1980s, the concept arose of using a single chromatographic system to carry out a range of separation modes, namely the unified chromatograph. Such an instrument (19) has been used (20) (Table 1.2) in the analysis of the complete range of products derived from petroleum, from gases to vacuum residues and polymers, with either open-tubular or packedcapillary columns, and gas, supercritical or liquid mobile phases.
Recently, Chester has described (21) how a consideration of the phase diagram of the mobile phase shows that a one-phase region (Figure 1.1) is available for the selection of the mobile phase parameters, and that the boundaries separating
Table 1.2 Application of unified chromatography in petroleum analysis (20)
Mode |
Sample |
Columna |
Detectionb |
GC |
Petroleum gases |
Packed capillary (ODS) |
FID |
|
Gasoline |
|
|
|
Kerosene |
Open tubular |
FID |
|
Diesel fuel |
|
|
SFC |
Petroleum wax |
|
FID |
|
Atmospheric and |
Open tubular |
|
|
vacuum residues |
|
|
|
Lube oil additives |
|
|
|
Aromatic fractions |
Packed capillary (ODS) |
UV, FID |
|
Lube oil additives |
Packed capillary (diol) |
FID |
GC – SFC |
Crude oil, etc. |
|
|
(sequential) |
Gasoline, diesel fuel in |
Open tubular |
FID |
|
lube oil |
|
|
HPLC and |
Aromatic fractions |
|
|
SFC/HPLC |
Polymers |
Packed capillary (SiO2) |
UV |
(sequential) |
|
|
|
|
|
|
|
aODS, octadecylsilyl silica.
bFID, flame-ionization detector.

6 |
Multidimensional Chromatography |
Figure 1.1 Phase diagram of the chromatographic mobile phase (after reference (21)), where the plane describes changing the composition and pressure at constant temperature.
individual techniques are totally arbitrary. By varying the pressure, temperature and composition, solute – mobile phase interactions can be varied so as to permit the elution of analytes ranging from permanent gases to ionic compounds; the dependence of the solute diffusion coefficient in the mobile phase on pressure, temperature and composition also influences mass transfer and therefore has an important bearing on the choice of an appropriate mobile phase.
The provinces of the common chromatographic separation modes are shown in Figure 1.1, with GC and HPLC practice corresponding to two of the areas; SFC with a single (here carbon dioxide) mobile phase is carried out on the front face of the diagram. In principle, however, any part of the phase diagram outside the two-phase region (shaded in Figure 1.1) may be employed. Figure 1.2 shows a microcolumn chromatogram obtained with simultaneous change of the pressure and composition of a carbon-dioxide mixture mobile phase, as indicated by the plane in Figure 1.1. Table 1.3 summarizes some of the uses of different regions of the phase diagram of the mobile phase.
1.3RESOLVING POWER OF CHROMATOGRAPHIC SYSTEMS
The peak capacity, n, of a single-column chromatographic system generating N theoretical plates is given by:
|
√ |
|
|
ln |
|
1 |
|
n |
N |
|
t2 |
(1.2) |
|||
4R |
|
t1 |
for a retention window from time t1 to t2. Some values of n for commonly used chromatographic separation methods are listed in Table 1.4, where it is immediately clear