
Multidimensional Chromatography
.pdf28 |
Multidimensional Chromatography |
2.4TRANSFER OF WATER-CONTAINING SOLVENT MIXTURES
A large number of samples to be analysed by gas chromatography have an aqueous matrix. Figure 2.1 shows that for the analysis of reversed-phase eluents both direct and indirect methods have been used. The direct injection of water-containing solvent mixtures in capillary GC would be attractive for accelerating an analysis but has many difficulties. The main drawbacks are as follows: the high boiling point and molar enthalpy of vaporising, thus requiring extensive solvent evaporation; due to its small molecular weight, water forms a very large volume of vapour per volume of liquid (about six time more than hexane), and elimination of this vapour volume via the column is tedious and time-consuming; condensed water destroys the deactivation of the precolumn due to the hydrolysis of siloxane bonds; the extremely high surface tension of water does not allow it to wet deactivated capillary surfaces, which thus makes water a poor solvent for the formation of the solvent film that is essential for solute trapping.
2.4.1DIRECT INJECTION BY USING A RETENTION GAP
On-column injection of large volumes of aqueous samples has achieved considerable attention in the field of on-line reversed phase LC–GC. The main problem in direct introduction of water, as mentioned above, is the poor wettability of the uncoated precolumns. In 1989, Grob and Li (20) tested several fused silica and glass precolumns deactivated by using different methods and concluded that the transfer of aqueous solvents by retention gap techniques was not achievable because it was impossible to find a precolumn that at the same time was both water-wettable and inert. This problem was tentatively solved by using an organic solvent with a higher boiling point than that of water. In fact, these same authors (21) investigated the wettability of phenyland cyanosilylated precolumns with mixtures of organic solvents and water, by using the retention gap technique for transferring water and mixtures of water with organic solvents. Their results demonstrated that, depending on the organic solvent being used, mixtures of such solvents with high concentrations of water still wet the precolumns (e.g. 70% water and 30% 1-propanol), although water did not evaporate together with the organic component. Azeotropically boiling mixtures, e.g. 28% water and 72% of 1-propanol, demonstrated that wettability of such precolumns is possible and thus allows the introduction of water by the retention gap technique.
2.4.2 DIRECT INJECTION BY USING CONCURRENT SOLVENT EVAPORATION
As mentioned above, concurrent solvent evaporation does not need good wettability of the solvent used for the LC–GC transfer. However, due to the large amount of vapour released and the high temperatures needed for concurrent solvent evaporation
Coupled HPLC with HRGC |
29 |
of water, this technique is limited to high-boiling analytes. As an illustration of this, the determination of atrazine in tap water is shown in Figure 2.9 (22). This method is based on enrichment of the atrazine from 10 ml of water on a small LC column packed with silica-C18, and desorption with methanol–water (60 : 40) 5% 1-propanol to the GC column by concurrent eluent evaporation using a loop-type interface. Both the retaining precolumn and the separation column were coated with Carbowax 20 M which had a very high retention for atrazine. This was necessary to obtain the high elution temperature required for atrazine. In fact, when transferring the LC fraction at 112 °C the atrazine peak was perfectly shaped only when eluted at about 250°C.
2.4.3 DIRECT INJECTION BY USING CONCURRENT SOLVENT EVAPORATION WITH A CO-SOLVENT
A partial solution to the problem of producing sharp peaks at low elution temperatures is to add a small amount of a higher-boiling co-solvent to the main solvent. As suggested by Grob and Muller (23, 24), butoxyethanol can be used as a suitable cosolvent for aqueous mixtures in such cases.
2.4.4 DIRECT INTRODUCTION OF WATER VIA A VAPORIZER CHAMBER/PRECOLUMN SOLVENT SPLIT/GAS
DISCHARGE INTERFACE
Recently, the direct introduction of water-containing eluents via a vaporizer chamber/precolumn solvent split/gas discharge interface has been reported (25, 26). Water and water-containing eluents were driven into a vaporizer chamber at 300°C by the LC pump (Figure 2.10). This high temperature permitted evaporation of water at a rate up to around 200 l/min. The vapours were then removed through a retaining precolumn and a early vapour exit, driven by the flow of carrier gas (discharge). The vaporizing chamber consisted of a 1 mm id glass tube, packed with a 2 cm plug of Carbofrit and internally coated with polyimide. Solvent /solute separation occurred in the retaining precolumn, and special attention was given to the oven temperature during the transfer, being held close to the temperature at which recondensation occurrs (the dew point). This method was successfully applied to the determination of phthalates in drinking water. Figure 2.11(a) shows a liquid chromatogram, obtained on a column packed with C-18 (5 m) bonded silica (1 cm 3 mm i.d.) of a water sample spiked with dibutyl phthalate (DBP) and diethylhexyl phthalate (DEHP). After sample enrichment, 10 ml of the fraction was transferred to the gas chromatograph, driven by the LC eluent (water/methanol 15:85) and by reducing the flow rate to 100 l/min. The LC–GC–MS(EI) chromatogram of the treated water containing 55 and 40 ng/l of DBP and DHEP, respectively is shown in Figure 2.11(b).
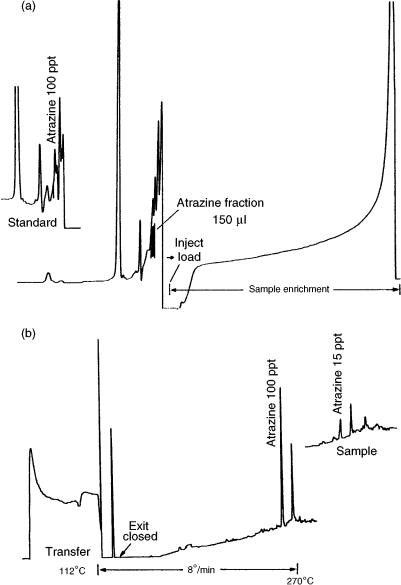
30 |
Multidimensional Chromatography |
Figure 2.9 (a) Liquid chromatogram (UV detection at 220 nm) obtained after initial sample enrichment (10 ml of water). At the point indicated, shortly before the content of the sample loop was completely transferred, the injection valve was switched, thus starting elution. The atrazine fraction is marked. On the left, part of the chromatogram of another tap water sample spiked with 100 ppt of atrazine is shown. (b) Gas chromatogram of tap water spiked with 100 ppt and 15 ppt of atrazine. Reprinted from Journal of Chromatography A, 473, K. Grob and Z. Li, ‘Coupled reversed-phase liquid chromatography – capillary gas chromatography for the determination of atrazine in water’, pp. 423 – 430, copyright 1989, with permission from Elsevier Science.
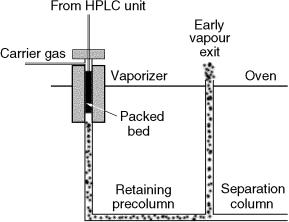
Coupled HPLC with HRGC |
31 |
Figure 2.10 Schematic representation of a vaporizing chamber/precolumn solvent split/gas discharge interface, where the vaporizer is packed and heated at a suitable temperature for solvent evaporation. The vapour exit can be positioned at the end of the retention gap.
2.5INDIRECT INTRODUCTION OF WATER
2.5.1SOLID PHASE EXTRACTION
A method for coupling reversed phase LC to GC without introducing water into the GC unit uses precolumns or solid-phase extraction (SPE) cartridges for the solutes enrichment, followed by drying and extraction with organic solvent. Normally, a large volume of water (1 ml) containing the organic compounds is passed through the precolumn. The organic compounds are then eluted by an organic solvent into the gas chromatograph, after flushing of the precolumn with nitrogen to remove the residual water. This method, mostly used by Brinkman and co-workers was first developed (27) by using an SPE cartridge (4 mm 1 mm id) inserted in a six-port valve and packed with C-18 bonded silica.
Subsequently, Vreuls and co-workers (28 – 31) used a small LC column installed on a 6- or 10-port valve. These columns usually had a length of 2 – 10 mm and an id of 1 – 4.6 mm (32). A large volume (1 – 10 ml) of aqueous sample containing the organic compounds is then passed and concentrated on a polymer-packed column. After several washings with water, the column must be dryed with nitrogen. The organic compounds are then eluted by using an organic solvent such as hexane, or ethyl acetate (e.g. 50 l) which is introduced on-line into the GC unit. Due to the large amount of organic solvent introduced into the gas chromatograph, large-volume introduction techniques are required, such as those discussed above. A typical on-line SPE – GC set-up is shown in Figure 2.12 (32).
Drying carried out with purging of a precolumn is time consuming, and an interesting alternative is the use of a drying cartridge positioned between the SPE and GC units. This small cartridge should be reconditioned during the GC run by heating. In
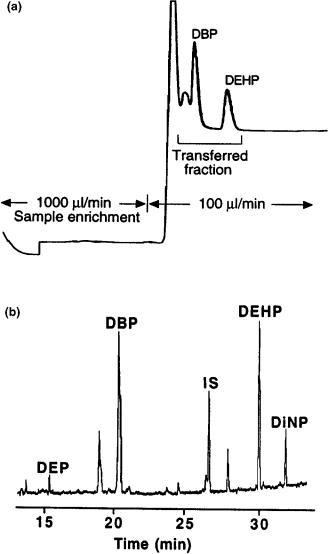
32 |
Multidimensional Chromatography |
Figure 2.11 (a) HPLC chromatogram obtained for a sample of drinking water spiked with dibutyl phthalate (DBP) and diethylhexyl phthalate (DEHP). (b) The LC–GC/MS chromatogram of the same sample after sample enrichment. Reprinted from Journal of High Resolution Chromatography, 20, T. Hyötyläinen et al., ‘Reversed phase HPLC coupled on-line to GC by the vaporizer/precolumn solvent split/gas discharge; analysis of phthalates in water’, pp. 410 – 416, with permission from Wiley-VCH.
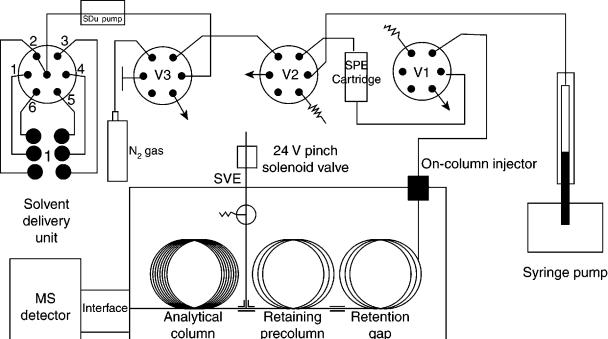
HRGC with HPLC Coupled
Figure 2.12 Schematic representation of an on-line SPE – GC system consisting of three switching valves (V1 – V3), two pumps (a solventdelivery unit (SDU) pump and a syringe pump) and a GC system equipped with a solvent-vapour exit (SVE), an MS instrument detector, a retention gap, a retaining precolumn and an analytical column. Reprinted from Journal of Chromatography, A 725, A. J. H. Louter et al., ‘Analysis of microcontaminants in aqueous samples by fully automated on-line solid-phase extraction – gas chromatography – mass selective detection’, pp. 67 – 83, copyright 1996, with permission from Elsevier Science.
33
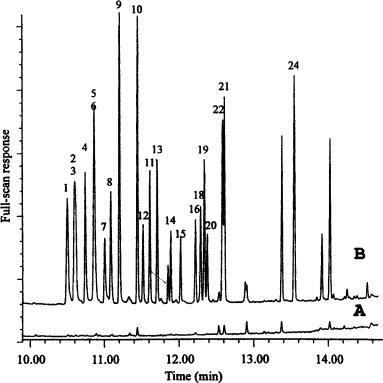
34 |
Multidimensional Chromatography |
a recent paper (33), three drying agents and four solvents were tested by using a mixture of 24 microcontaminants ranging widely in polarity and volatility. Silica was preferred among the drying agents investigated (sodium sulfate, silica and molecular sieves), while methyl acetate was the preferred solvent after examining pentane, hexane, ethyl acetate and methyl acetate. In fact, Figure 2.13 shows the on-line SPE- drying–GC–MS chromatogram of tap water spiked with 24 pollutants at low-ng/l detection levels.
Figure 2.13 On-line SPE – GC – MS (full scan; m/z 35 – 435) chromatograms of 10 ml of (Amsterdam) tap water without (A) and with (B) spiking at the 0.5 g/l level. Peak assignment: 1, 3,4-dichlorobenzene; 2, dimethylphthalate; 3, 1,3-dinitrobenzene; 4, 4-butoxyphenol; 5, acenaphthene; 6, 3-nitroanalinine; 7, 1-naphthenelol; 8, pentachlorobenzene; 9, 2,5- diethoxyaniline; 10, diethylphthalate; 11, 1-nitrophthalene; 12, 1,2,4,6-bis-O-(1-methylethyli- dine)-a-L-sorbofuranose; 13, ributylphosphate; 14, trifluralin; 15, 1,4-dibutoxybenzene; 16, hexachlorobenzene; 17, dimethoate; 18, simazine; 19, atrazine; 20, trichloroethylphosphate; 21, phenantrene, 22, diazinon (internal standard) 23, caffeine; 24, metolachlor. Reprinted from Journal of High Resolution Chromatography, 21, T. Hankemeier et al., ‘Use of a drying cartridge in on-line solid-phase extraction – gas chromatography – mass spectrometrys, pp. 450 – 456, 1998, with permission from Wiley-VCH.
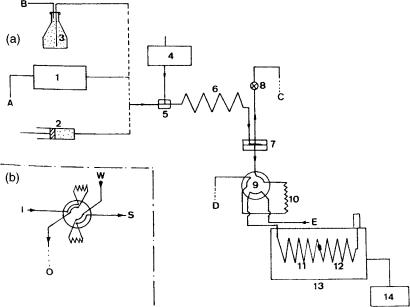
Coupled HPLC with HRGC |
35 |
2.5.2LIQUID–LIQUID EXTRACTION
This method relies on the on-line extraction of an organic compound from an aqueous sample by using an organic solvent. In automated liquid–liquid extraction (LLE), the solvent (organic) is injected via a small segmentor into the water sample, which flows through a small-diameter fused silica tube. Phase separation is achieved by using a semipermeable PTFE membrane (34 – 36) or a sandwich-type phase separator. For the first case (34), the configuration adopted is illustrated in Figure 2.14. The water sample was delivered by either an HPLC pump, syringe or a pressurized flask, and then extracted with n-pentane. Separation of the two phases was achieved by using a membrane separator (Figure 2.15), based on a semipermeable PTFE membrane, which has different wetting characteristic for the phases. This system was used to analyse volatile organic trace compounds, as shown in Figure 2.16 where a water sample was spiked with 200 ppb of a naphtha fraction (34).
Figure 2.14 Schematic representation of the set-up used for continuous flow extraction with on-line capillary GC: (A) 1 and 4 HPLC pumps; 2, syringe pump; 3, pressurized flask with water sample; 5, segmentor; 6, fused-silica capillary tube; 7, phase separator; 8, needle valve; 9, six-port valve; 10, sample loop; 11, empty precolumn; 12, GC column; 13, GC oven; 14, recorder/integrator; A, water sample; B, pressurized nitrogen, C, extracted water; D, extracted drain; E, carrier gas: (B) I, sample; O, sample drain; W, clean water stream; S, segmentor. Reprinted from Journal of Chromatography, A 330, J. Roeraade, ‘Automated monitoring of organic trace components in water. I. Continuous flow extraction together with on-line capillary gas chromatography’, pp. 263 – 274, copyright 1985, with permission from Elsevier Science.
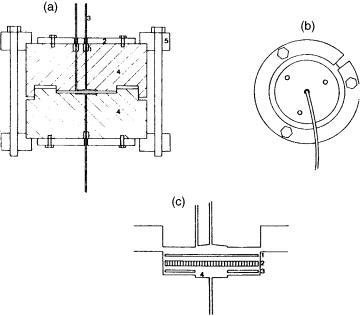
36 |
Multidimensional Chromatography |
Figure 2.15 Schematic representation of the phase separator: (A) 1, cavity with silicone rubber O-ring; 2, stainless-steel compression plate; 3, fused-silica tubing; 4, stainless-steel body; 5, aluminium ring: (B) bottom view of the separator (top view when solvents with a lower density than that of water are employed): (C) enlarged view of the separator cavities; 1, Fluoropore membrane; 2, stainless-steel screen; 3, Fluoropore washer; 4, cavity for the organic phase (diameter 4 mm; depth 0.1 mm). Reprinted from Journal of Chromatography A, 330, J. Roeraade, ‘Automated monitoring of organic trace components in water. I. Continuous flow extraction together with on-line capillary gas chromatography’, pp. 263 – 274, copyright 1985, with permission from Elsevier Science.
Another approach is where membranes are not used in the construction material of the sandwich-phase separator (36). In this configuration, water was pumped through the system by using a membrane pump (1 ml/min) with the organic phase (isooctane) being delivered by a second membrane pump (again at a rate of 1 ml/min). The extraction coil was a 6.4 m long PTFE tube with phase separation being accomplished by the use of a sandwich-phase separator (Figure 2.17) (36,37). with this arrangement, 100 l of isooctane could be injected by fully concurrent solvent evaporation. By using such a system the determination of 0.1 pg/l of hexachlorocyclohexanes in water was possible (Figure 2.18).
A similar on-line LLE system, equipped with an on-line derivatization system, has also been reported. Brinkman and co-workers, for example, have presented a complete automated on-line system that allows both the LLE and alkylation of organic acids (38). More recently, this same group have reported the coupling of
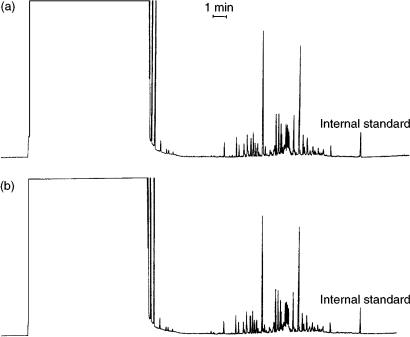
Coupled HPLC with HRGC |
37 |
Figure 2.16 Chromatograms of a pentane extract of a water sample containing 200 ppb of a naphtha fraction: (a) sample extracted by using a continuous flow system, where a pressurized bottle was employed as the sample-delivery system; (b) batch-extracted sample. Reprinted from Journal of Chromatography, A 330, J. Roeraade, ‘Automated monitoring of organic trace components in water. I. Continuous flow extraction together with on-line capillary gas chromatography’, pp. 263 – 274, copyrigth 1985, with permission from Elsevier Science.
such a system with an atomic emission detector (AED) for the analysis of nitrogenchlorineand sulfur-containing pesticides in aqueous samples (39), as shown in Figure 2.19.
2.5.3OPEN TUBULAR TRAPPING
The analysis of organic compounds from aqueous samples is also possible by using open tubular trapping (OTT) columns (13, 40 – 42). The extraction step involves sorption of the analytes from water (40) into the stationary phase of an open tube (5 m 0.53 mm id) with a film of CP-Sil-5CB (5 m film thick). Removal of water is achieved by purging the trap with nitrogen, and desorption of the analytes with an organic solvent (75 l). Solvent elimination prior to transfer to the GC column is carried out by using a PTV injector and a multidimensional system (Figure 2.20).