
Astruc D. - Modern arene chemistry (2002)(en)
.pdf
References 213
ization can be controlled, providing molecular photoswitches; absorptions up to the near-IR region are obtained upon extending the linear conjugation, cyclic cross-conjugation in expanded radialenes is enforced by donor groups; the optical gap of PTA oligomers is decreased; strong fluorescence behavior can be introduced in PTAs, and the e ective conjugation length in PTAs can be adjusted by changing the donor strength of the end groups.
Abbreviations
Aacceptor
Ac |
acetyl |
Bu |
butyl |
CT |
charge-transfer |
Ddonor
DEE |
trans-1,2-diethynylethene |
DHA |
dihydroazulene |
DMA |
dimethylaniline |
ECL |
e ective conjugation length |
Et |
ethyl |
Fc |
ferrocene |
fs |
fused silica |
IR |
infrared |
Me |
methyl |
NLO |
nonlinear optics |
PA |
polyacetylene |
Ph |
phenyl |
PDA |
poly(diacetylene) |
Pr |
propyl |
PTA |
poly(triacetylene) |
TEE |
tetraethynylethene |
UV |
ultraviolet |
TICT |
twisted intramolecular charge-transfer |
TMEDA |
N,N,N 0,N 0-tetramethylethylenediamine |
VHF |
vinylheptafulvene |
vis |
visible |
References
1 For reviews on acetylenic molecular |
Curr. Chem. 1999, 201, 131–161; e) F. |
sca olding, see: a) F. Diederich, Nature |
Diederich, L. Gobbi, Top. Curr. Chem. |
1994, 369, 199–207; b) L. T. Scott, M. J. |
1999, 201, 43–79; f ) P. Siemsen, R. C. |
Cooney, in Modern Acetylene Chemistry |
Livingston, F. Diederich, Angew. Chem. |
(Eds.: P. J. Stang, F. Diederich), VCH, |
2000, 112, 2740–2767; Angew. Chem. Int. |
Weinheim, 1995, pp. 321–351; c) U. H. F. |
Ed. 2000, 39, 2632–2657. |
Bunz, Y. Rubin, Y. Tobe, Chem. Soc. Rev. |
2 F. Diederich, Chem. Commun. 2001, 219– |
1999, 28, 107–119; d) U. H. F. Bunz, Top. |
227. |

214 6 Functional Conjugated Materials for Optonics and Electronics by Tetraethynylethene Molecular Scaffolding
3K. Sonogashira, in Metal-Catalyzed CrossCoupling Reactions (Eds.: F. Diederich,
P. J. Stang), Wiley-VCH, Weinheim, 1998, pp. 203–229.
4Recent reviews: a) R. R. Tykwinski, F. Diederich, Liebigs Ann./Recueil 1997, 649–661; b) M. B. Nielsen, F. Diederich,
Synlett 2002, 544–552.
5C. Glaser, Ber. Dtsch. Chem. Ges. 1869, 2, 422–424.
6G. Eglinton, A. R. Galbraith, Chem. Ind. (London) 1956, 737–738.
7A. S. Hay, J. Org. Chem. 1962, 27, 3320– 3321.
8 a) W. Chodkiewicz, P. Cadiot, C. R. Hebd. Seances Acad. Sci. 1955, 241, 1055– 1057; b) W. Chodkiewicz, Ann. Chim. (Paris) 1957, 2, 819–869.
9 H. Hopf, M. Kreutzer, P. G. Jones,
Chem. Ber. 1991, 124, 1471–1475.
10A. Hoekstra, A. Vos, Acta Cryst. B 1975,
31, 1716–1721.
11D. Philp, V. Gramlich, P. Seiler, F. Diederich, J. Chem. Soc., Perkin Trans. 2
1995, 875–886.
12a) C. Bosshard, R. Spreiter, P. Gu¨nter, R. R. Tykwinski, M. Schreiber, F. Diederich, Adv. Mater. 1996, 8, 231–234; b) R. R. Tykwinski, M. Schreiber, V. Gramlich, P. Seiler, F. Diederich, Adv. Mater. 1996, 8, 226–231; c) R. Spreiter, C. Bosshard, G. Kno¨pfle, P. Gu¨nter, R. R. Tykwinski, M. Schreiber, F. Diederich,
J. Phys. Chem. B. 1998, 102, 4451–4465; d)
R. R. Tykwinski, U. Gubler, R. E. Martin, F. Diederich, C. Bosshard, P. Gu¨nter, J. Phys. Chem. B. 1998, 102,
4451–4465; e) U. Gubler, R. Spreiter, C. Bosshard, P. Gu¨nter, R. R. Tykwinski, F. Diederich, Appl. Phys. Lett. 1998, 73, 2396–2398; f ) C. Bosshard, in Nonlinear Optical E ects and Materials (Ed.:
P. Gu¨nter), Springer Verlag, Berlin, 2000, pp. 7–161; g) U. Gubler, C. Bosshard, Phys. Rev. B 2000, 61, 10702– 10710.
13L.-T. Cheng, W. Tam, S. R. Marder, A. E. Stiegman, G. Rikken, C. W. Spangler, J. Phys. Chem. 1991, 95, 10643–10652.
14C. W. Spangler, K. O. Havelka, M. W. Becker, T. A. Kelleher, L.-T. Cheng,
Proc. SPIE – Int. Soc. Opt. Eng. 1991, 1560, 139–147.
15a) J. Messier, F. Kajzar, C. Sentein, M.
Barzoukas, J. Zyss, M. BlanchardDesce, J.-M. Lehn, Nonlinear Opt. 1992, 2, 53–70; b) G. Puccetti, M. BlanchardDesce, I. Ledoux, J.-M. Lehn, J. Zyss, J. Phys. Chem. 1993, 97, 9385–9391; c) M. Blanchard-Desce, J.-M. Lehn, M. Barzoukas, C. Runser, A. Fort, G. Puccetti, I. Ledoux, J. Zyss, Nonlinear Opt. 1995, 10, 23–36.
16a) A. F. Garito, J. R. Heflin, K. Y. Wong, O. Zamani-Khamiri, in Organic Materials for Nonlinear Optics (Eds.: R. A. Hann, D. Bloor), The Royal Society of Chemistry, London, 1989, pp. 16–27; b)
J.W. Wu, J. R. Heflin, R. A. Norwood,
K.Y. Wong, O. Zamani-Khamiri, A. F. Garito, P. Kalyanaraman, J. Sounik, J. Opt. Soc. Am. B 1989, 6, 707–720.
17S. R. Marder, W. E. Torruellas, M. Blanchard-Desce, V. Ricci, G. I. Stegeman, S. Gilmour, J.-L. Bre´das, J. Li, G. U. Bublitz, S. G. Boxer, Science (Washington D.C.) 1997, 276, 1233–1236.
18a) I. D. L. Albert, J. O. Morley, D. Pugh,
J.Phys. Chem. 1995, 99, 8024–8032; b) V.
Keshari, W. Wijekoon, P. N. Prasad, S. P. Karna, J. Phys. Chem. 1995, 99, 9045– 9050; c) P. R. Varanasi, A. K.-Y. Jen, J.
Chandrasekhar, I. N. N. Namboothiri, A. Rathna, J. Am. Chem. Soc. 1996, 118, 12443–12448; d) I. D. L. Albert, T. J.
Marks, M. A. Ratner, J. Am. Chem. Soc.
1997, 119, 6575–6582.
19a) L.-T. Cheng, W. Tam, S. R. Marder,
A.E. Stiegman, G. Rikken, C. W. Spangler, J. Phys. Chem. 1991, 95, 10643– 10652; b) S. P. Karna, Y. Zhang, M. Samoc, P. N. Prasad, B. A. Reinhardt,
A.G. Dillard, J. Chem. Phys. 1993, 99, 9984–9993; c) V. P. Rao, A. K.-Y. Jen, K.
Y.Wong, K. J. Drost, J. Chem. Soc.,
Chem. Commun. 1993, 1118–1120; d) A. K.-Y. Jen, V. P. Rao, K. Y. Wong, K. J. Drost, J. Chem. Soc., Chem. Commun.
1993, 90–92; e) K. Y. Wong, A. K.-Y. Jen,
V.P. Rao, K. J. Drost, J. Chem. Phys.
1994, 100, 6818–6825; f ) V. P. Rao, K. Y. Wong, A. K.-Y. Jen, K. J. Drost, Chem. Mater. 1994, 6, 2210–2212; g) P. Boldt,
G.Bourhill, C. Bra¨uchle, Y. Jim, R. Kammler, C. Mu¨ller, J. Rase, J. Wichern, Chem. Commun. 1996, 793– 795; h) S.-S. P. Chou, D.-J. Sun, H.-C. Lin, P.-K. Yang, Chem. Commun. 1996,
|
|
|
References |
215 |
|
|
|
|
|
|
1045–1046; i) V. P. Rao, A. K.-Y. Jen, Y. |
|
Grabowski, A. Krwoczynski, W. |
|
|
Cai, Chem. Commun. 1996, 1237–1238; |
|
Ku¨hnle, J. Lumin. 1976, 12/13, 877–885; |
|
|
j) C. Z. Cai, I. Liakatas, M.-S. Wong, |
|
c) Z. R. Grabowski, K. Rotkiewicz, A. |
|
|
M. Bo¨sch, C. Bosshard, P. Gu¨nter, S. |
|
Siemiarczuk, J. Lumin. 1979, 18/19, 420– |
|
|
Concilio, N. Tirelli, U. W. Suter, Org. |
|
424; d) Z. R. Grabowski, K. Rotkiewicz, |
|
|
Lett. 1999, 1, 1847–1849. |
|
A. Siemiarczuk, D. J. Cowley, W. |
|
20 |
a) M.-T. Zhao, B. P. Singh, P. N. Prasad, |
|
Baumann, Nouv. J. Chim. 1979, 3, 443– |
|
|
J. Chem. Phys. 1988, 89, 5535–5541; b) |
|
454. |
|
|
V. P. Rao, A. K.-Y. Jen, K. Y. Wong, K. J. |
27 |
A. Hilger, J. P. Gisselbrecht, R. R. |
|
|
Drost, Tetrahedron Lett. 1993, 34, 1747– |
|
Tykwinski, C. Boudon, M. Schreiber, |
|
|
1750; c) K. Y. Wong, A. K.-Y. Jen, V. P. |
|
R. E. Martin, H. P. Lu¨thi, M. Gross, F. |
|
|
Rao, Phys. Rev. A 1994, 49, 3077–3080. |
|
Diederich, J. Am. Chem. Soc. 1997, 119, |
|
21 |
For examples of other cis-trans |
|
2069–2078. |
|
|
photoisomerizations about a central |
28 |
R. E. Martin, J. Bartek, F. Diederich, R. |
|
|
carbon–carbon double bond, see: a) D. |
|
R. Tykwinski, E. C. Meister, A. Hilger, |
|
|
Schulte-Frohlinde, H. Go¨rner, Pure |
|
H. P. Lu¨thi, J. Chem. Soc., Perkin Trans. 2 |
|
|
Appl. Chem. 1979, 51, 279–297; b) D. H. |
|
1998, 233–241. |
|
|
Waldeck, Chem. Rev. 1991, 91, 415–436; |
29 |
a) A. M. Boldi, F. Diederich, Angew. |
|
|
c) H. Meier, Angew. Chem. 1992, 104, |
|
Chem. 1994, 106, 482–485; Angew. Chem. |
|
|
1425–1446; Angew. Chem. Int. Ed. Engl. |
|
Int. Ed. Engl. 1994, 33, 468–471; b) J. |
|
|
1992, 31, 1399–1420; c) B. L. Feringa, W. |
|
Anthony, A. M. Boldi, C. Boudon, J.-P. |
|
|
F. Jager, B. de Lange, Tetrahedron 1993, |
|
Gisselbrecht, M. Gross, P. Seiler, C. B. |
|
|
49, 8267–8310; d) W. F. Jager, J. C. de |
|
Knobler, F. Diederich, Helv. Chim. Acta |
|
|
Jong, B. de Lange, N. P. M. Huck, A. |
|
1995, 78, 797–817; c) M. Schreiber, R. R. |
|
|
Meetsma, B. L. Feringa, Angew. Chem. |
|
Tykwinski, F. Diederich, R. Spreiter, U. |
|
|
1995, 107, 346–349; Angew. Chem. Int. Ed. |
|
Gubler, C. Bosshard, I. Poberaj, P. |
|
|
Engl. 1995, 34, 348–350; e) B. L. Feringa, |
|
Gu¨nter, C. Boudon, J.-P. Gisselbrecht, |
|
|
N. P. M. Huck, A. M. Schoevaars, Adv. |
|
M. Gross, U. Jonas, H. Ringsdorf, Adv. |
|
|
Mater. 1996, 8, 681–684; f ) R. Ballardini, |
|
Mater. 1997, 9, 339–343; d) M. B. Nielsen, |
|
|
V. Balzani, J. Becher, A. Di Fabio, M. T. |
|
M. Schreiber, Y. G. Baek, P. Seiler, S. |
|
|
Gandolfi, G. Mattersteig, M. B. |
|
Lecomte, C. Boudon, R. R. Tykwinski, |
|
|
Nielsen, F. M. Raymo, S. J. Rowan, J. F. |
|
J.-P. Gisselbrecht, V. Gramlich, P. J. |
|
|
Stoddart, A. J. P. White, D. J. Williams, |
|
Skinner, C. Bosshard, P. Gu¨nter, M. |
|
|
J. Org. Chem. 2000, 65, 4120–4126. |
|
Gross, F. Diederich, Chem. Eur. J. 2001, |
|
22 |
R. R. Tykwinski, M. Schreiber, R. P. |
|
7, 3263–3280. |
|
|
Carlo´n, F. Diederich, V. Gramlich, |
30 |
a) J. Anthony, C. B. Knobler, F. |
|
|
Helv. Chim. Acta 1996, 79, 2249–2281. |
|
Diederich, Angew. Chem. 1993, 105, |
|
23 |
a) L. Gobbi, P. Seiler, F. Diederich, |
|
437–440; Angew. Chem. Int. Ed. Engl. |
|
|
Angew. Chem. 1999, 111, 737–740; Angew. |
|
1993, 32, 406–409; b) C. Boudon, J.-P. |
|
|
Chem. Int. Ed. 1999, 38, 674–678; b) L. |
|
Gisselbrecht, M. Gross, J. Anthony, |
|
|
Gobbi, P. Seiler, F. Diederich, V. |
|
A. M. Boldi, R. Faust, T. Lange, D. |
|
|
Gramlich, Helv. Chim. Acta 2000, 83, |
|
Philp, J.-D. van Loon, F. Diederich, J. |
|
|
1711–1723; c) L. Gobbi, P. Seiler, F. |
|
Electroanal. Chem. 1995, 394, 187–197. |
|
|
Diederich, V. Gramlich, C. Boudon, |
31 |
W. H. Okamura, F. Sondheimer, J. Am. |
|
|
J.-P. Gisselbrecht, M. Gross, Helv. Chim. |
|
Chem. Soc. 1967, 89, 5991–5992. |
|
|
Acta 2001, 84, 743–777. |
32 |
a) R. Wolovsky, F. Sondheimer, J. Am. |
|
24 |
S. Gierisch, J. Daub, Chem. Ber. 1989, |
|
Chem. Soc. 1965, 87, 5720–5727; b) F. |
|
|
122, 69–75. |
|
Sondheimer, R. Wolovsky, P. J. |
|
25 |
L. Gobbi, N. Elmaci, H. P. Lu¨thi, F. |
|
Garratt, I. C. Calder, J. Am. Chem. Soc. |
|
|
Diederich, ChemPhysChem 2001, 2, 423– |
|
1966, 88, 2610. |
|
|
433. |
33 |
K. G. Untch, D. C. Wysocki, J. Am. |
|
26 |
a) K. Rotkiewicz, K. H. Grellmann, |
|
Chem. Soc. 1966, 88, 2608–2610. |
|
|
Z. R. Grabowski, Chem. Phys. Lett. 1973, |
34 |
For a review on radialenes, see: H. Hopf, |
|
|
19, 315–318; b) K. Rotkiewicz, Z. R. |
|
G. Maas, Angew. Chem. 1992, 104, 953– |

216 6 Functional Conjugated Materials for Optonics and Electronics by Tetraethynylethene Molecular Scaffolding
977; Angew. Chem. Int. Ed. Engl. 1992, 31, 931–954.
35a) A. de Meijere, S. Kozhushkov, T. Haumann, R. Boese, C. Puls, M. J. Cooney,
L.T. Scott, Chem. Eur. J. 1995, 1, 124–131; b) M. Brake, V. Enkelmann, U. H. F. Bunz, J. Org. Chem. 1996, 61, 1190–1191.
36R. Faust, F. Diederich, V. Gramlich,
P.Seiler, Chem. Eur. J. 1995, 1, 111–117.
37a) D. T. McQuade, A. E. Pullen, T. M. Swager, Chem. Rev. 2000, 100, 2537–2574; b) U. H. F. Bunz, Chem. Rev. 2000, 100, 1605–1644.
38a) C. K. Chiang, C. R. Fincher, Jr., Y.
W.Park, A. J. Heeger, H. Shirakawa, E.
J.Louis, S. C. Gau, A. G. MacDiarmid,
Phys. Rev. Lett. 1977, 39, 1098–1101; b) H. Shirakawa, Angew. Chem. 2001, 113, 2642–2648; Angew. Chem. Int. Ed. 2001,
40, 2574–2580; c) A. G. MacDiarmid,
Angew. Chem. 2001, 113, 2649–2659; Angew. Chem. Int. Ed. 2001, 40, 2581– 2590; d) A. J. Heeger, Angew. Chem. 2001,
113, 2660–2682; Angew. Chem. Int. Ed.
2001, 40, 2591–2611.
39M. Schreiber, J. Anthony, F. Diederich, M. E. Spahr, R. Nesper, M. Hubrich, F. Bommeli, L. Degiorgi, P. Wachter, P. Kaatz, C. Bosshard, P. Gu¨nter, M. Colussi, U. W. Suter, C. Boudon, J.-P. Gisselbrecht, M. Gross,
Adv. Mater. 1994, 6, 786–790.
40Recently, Fowler and co-workers reported a very elegant PTA synthesis by topo-
chemically controlled polymerization of hexa-1,3,5-triynes in the crystal: J. Xiao, M. Yang, J. W. Lauher, F. W. Fowler,
Angew. Chem. 2000, 112, 2216–2219; Angew. Chem. Int. Ed. 2000, 39, 2132– 2135.
41C. J. Hawker, J. M. J. Fre´chet, J. Am. Chem. Soc. 1990, 112, 7638–7647.
42A. P. H. J. Schenning, J.-D. Arndt, M. Ito, A. Stoddart, M. Schreiber, P. Siemsen, R. E. Martin, C. Boudon, J.-P. Gisselbrecht, M. Gross, V. Gramlich,
F.Diederich, Helv. Chim. Acta 2001, 84, 296–334.
43a) J. Wytko, V. Berl, M. McLaughlin,
R.R. Tykwinski, M. Schreiber, F. Diederich, C. Boudon, J.-P. Gisselbrecht, M. Gross, Helv. Chim. Acta
1998, 81, 1964–1977; b) E. M. Maya, P. Vazquez, T. Torres, L. Gobbi, F. Diederich, S. Pyo, L. Echegoyen, J. Org. Chem. 2000, 65, 823–830; c) R. E. Martin, J. A. Wytko, F. Diederich, C. Boudon, J.-P. Gisselbrecht, M. Gross, Helv. Chim. Acta 1999, 82, 1470–1485; d) M. J. Edelmann, S. Odermatt, F. Diederich,
Chimia 2001, 55, 1–11.
44P. Siemsen, U. Gubler, C. Bosshard, P. Gu¨nter, F. Diederich, Chem. Eur. J.
2001, 7, 1333–1341.
45R. E. Martin, U. Gubler, C. Boudon, C. Bosshard, J.-P. Gisselbrecht, P. Gu¨nter, M. Gross, F. Diederich, Chem. Eur. J. 2000, 6, 4400–4412.

217
7
The ADIMET Reaction: Synthesis and Properties of Poly(dialkylparaphenyleneethynylene)s
Uwe H. F. Bunz
Abstract
A mixture of molybdenum hexacarbonyl and 4-chlorophenol is e ective in performing alkyne metathesis of dipropynylated dialkylbenzenes. Alkyne metathesis of these precursors leads to the clean formation of dialkyl poly( paraphenyleneethynylene)s (PPEs) in high yield and with high molecular weights. This facile yet e ective access to the PPEs has allowed study of their spectroscopic, structural, and thermal properties. While PPEs have been made before, the dialkyl-PPEs turned out to have particularly interesting optical and liquidcrystalline properties that can be explained in terms of the conformation of the main chains. The PPEs have also been utilized to construct light-emitting diodes and other semiconductor devices. This chapter discusses the interplay of structure, chromicity, and electronic properties of the dialkyl-PPEs.
7.1
Introduction
7.1.1
Scope and Coverage of this Review
This contribution describes the synthesis and properties of organic alkyne-bridged materials that have been made by alkyne metathesis or by Acyclic Diyne Metathesis (ADIMET). This review covers mostly poly(aryleneethynylene)s made at the author’s laboratory in South Carolina. A comprehensive review covering poly(aryleneethynylene)s listing important contributions in adjacent areas has appeared [1, 2]. This chapter discusses the synthesis and properties of dialkyl-poly(paraphenyleneethynylene)s (dialkyl-PPEs) in relation to the concept of ADIMET with simple catalyst systems.
7.1.2
Historical Perspective
The metathesis of carbon–carbon multiple bonds has ‘‘exploded’’ in the last ten years. In particular, alkene metathesis, that is the transition metal promoted 1,2-permutation of
Modern Arene Chemistry. Edited by Didier Astruc
Copyright 8 2002 WILEY-VCH Verlag GmbH & Co. KGaA, Weinheim ISBN: 3-527-30489-4
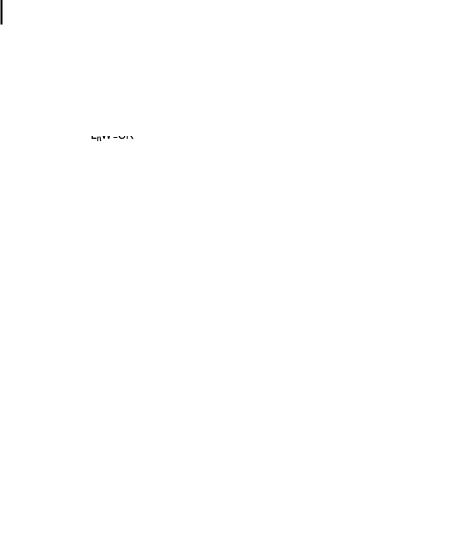
2187 The ADIMET Reaction: Synthesis and Properties of Poly(dialkylparaphenyleneethynylene)s
substituents in alkenes (Scheme 1), has made great strides since the commercialization of Grubbs’ and Schrock’s ruthenium and molybdenum alkylidenes. Herrmann discovered that imidazolium ligands boost the reactivity of the Grubbs catalysts. Alkene metathesis has been applied in all fields of organic and materials chemistry, and has been covered by excellent review articles [3, 4].
Scheme 1
On the other hand, alkyne metathesis (Scheme 1, 2), is the permutation of substituents in alkynes in a process that involves the transition metal catalyzed scission of the carbon– carbon triple bond [5]. The first example of this reaction in homogeneous solution was found by Mortreux [6]; when he heated a mixture of diphenylacetylene, ditolylacetylene, Mo(CO)6, and catechol in a high-pressure reactor, he observed the formation of the mixed phenyltolylacetylene 3 in the reaction mixture (Scheme 2). Although this reaction set-up is simple, Mortreux did not exploit this attractive system for the preparation of organic target molecules. He did not address the question of the catalytically active species, even though several more interesting papers covering the topic appeared in the literature [5].
Scheme 2
In the early 1980s, Schrock prepared a series of tungstenand molybdenum-based carbyne complexes, and demonstrated that they are viable catalysts for performing stoichiometric and catalytic alkyne metathesis [7]. With the defined carbyne complexes, he laid the foundation for the mechanistic understanding of alkyne metathesis, and was the first to demonstrate that vinyl-substituted carbyne complexes are stable [8] and that alkyne metathesis could be performed in the presence of CbC double bonds.
From a mechanistic point of view (Scheme 3), a transition metal carbyne undergoes a ½2þ2& cycloaddition with an alkyne to form a metallacyclobutadiene as an intermediate, or possibly as a transition state. The four-membered ring then cycloreverts, expelling a disubstituted alkyne with regeneration of a catalytically active carbyne complex. In a subsequent
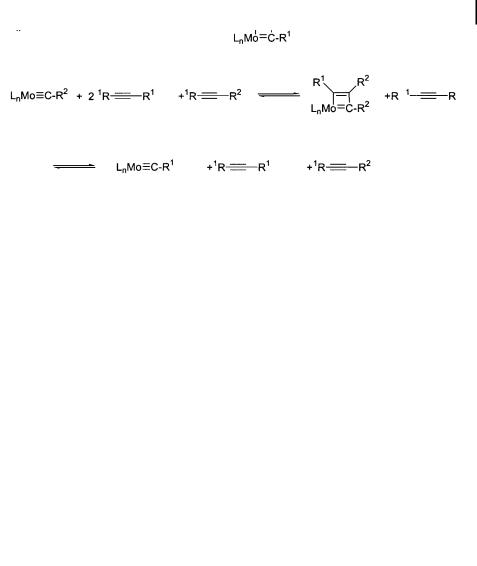
7.1 Introduction 219
Scheme 3
reaction, another ½2þ2& cycloaddition to give a metallacyclobutadiene occurs. Cycloreversion again regenerates the catalytically active species, and ejects the final product of the metathesis.
Schrock and Krouse utilized alkyne metathesis to ring-open cyclooctyne 4 [9]. They obtained a polycyclooctynamer 5. This ROMP is the first known application of a carbyne complex in polymer science. Bazan showed in 1994 elegantly that alkyne metathesis of siliconcontaining diynes 6 with tungsten carbynes of the Schrock-type furnishes conjugated polymers 7 [10]. However, while both of these seminal papers were of high importance and exciting, the results lacked their well-deserved impact (Scheme 4).
Scheme 4
Further interest in the use of alkyne metathesis in polymer science arose through a collaborative e ort of Bunz and Mu¨llen, exploring the synthesis of poly(paraphenyleneethynylene)s (PPEs) by Pd-catalyzed couplings [11]. The PPEs that were isolated were of relatively low molecular weight and had a significant amount of defects, a consequence inherent to the Pd methodology. The presence of diyne units, the occurrence of phosphonium end groups, and dehalogenation generally plague this – per se valuable and generally applicable – synthetic approach. Alkyne metathesis is proposed as an alternative route to PPEs that may circumvent the problems associated with Pd catalysis.
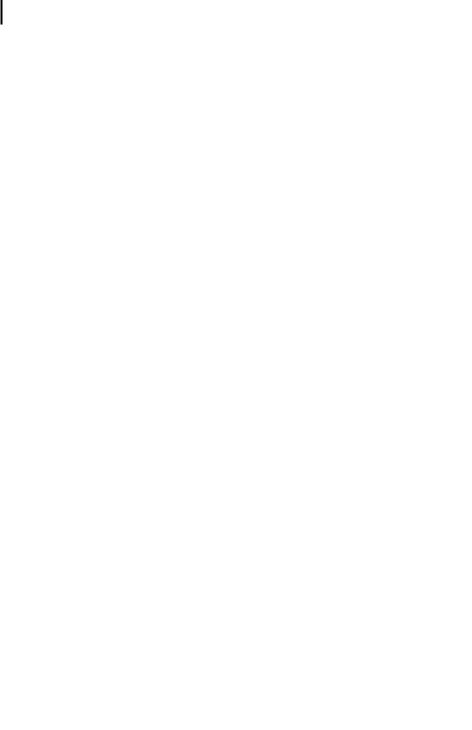
220 7 The ADIMET Reaction: Synthesis and Properties of Poly(dialkylparaphenyleneethynylene)s
In 1995, Mori revived alkyne metathesis with simple Mo(CO)6-based catalyst systems, and successfully prepared a series of diaryland arylalkylacetylenes (Scheme 5) [12]. The yields of the dimerization reactions were not particularly high. The simplicity of the method utilizing o -the-shelf catalyst precursors in combination with technical quality solvents made these systems potentially valuable for the synthesis of novel aromatic oligomers and polymers (Scheme 5). Mori o ered an alternative mechanistic picture as to how alkyne metathesis might work in these simple catalyst systems, which invoked a transient molybdenacyclopentadiene 9a; the classic interpretation of alkyne metathesis according to Schrock involves an intermediate 9b as shown in Scheme 5.
Scheme 5
7.2
Syntheses
7.2.1
PPEs by Acyclic Diyne Metathesis (ADIMET) Utilizing Schrock’s Tungsten Carbyne Complex 11
The first conjugated polymer to be made by the ADIMET process was dihexyl-PPE (12a) [13]. Reaction of 1,4-dipropynyl-2,5-dihexylbenzene (10a) with (tBuO)3WcCtBu (11) at 80 C in vacuo in a high boiling solvent furnished dihexyl-PPE 12a as a yellow powder in 80 % yield and with a degree of polymerization (Pn) of 94 repeat units (Scheme 6). The material was characterized by NMR spectroscopy, and did not show any defects that could be attributed to
Scheme 6
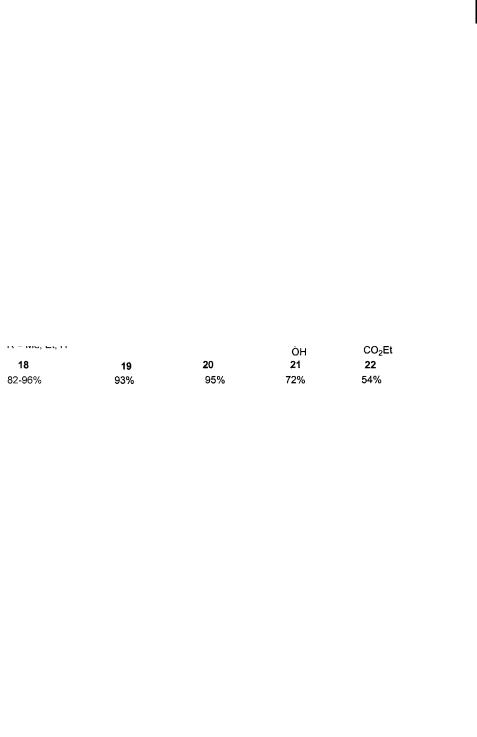
7.2 Syntheses 221
cross-linking, electrophilic addition of aryl oxides, or partial reduction. In an extension of this approach, 1,4-dipropynyl-2,5-diphenylbenzene 10b and 1,3-dipropynylbenzene were metathesized to give poly(aryleneethynylene)s in good yields but with a relatively low degree of polymerization. The successful performance of alkyne metathesis inspired Fu¨rstner [14] to utilize ADIMET to close large rings in a clever exploitation of alkyne metathesis in natural product synthesis. While the defined carbyne complex 11 is successful in the synthesis of PPEs, this alkylidyne is not commercially available. It has to be synthesized in a multistep procedure utilizing glove-box techniques, a task that the preparative by working organic chemist wants to avoid! As a consequence, it was of great importance to explore whether the simple in situ catalysts formed from Mo(CO)6 and 4-chlorophenol could be used for the synthesis of PPEs 12 and other target molecules.
7.2.2
Synthesis of Diarylalkynes Utilizing the Mori System
The low yields of the Mori dimerization presented a challenge for the utilization of the simple catalyst systems [12]: optimization by increasing the reaction temperature from 105 to 130–150 C combined with the slow introduction of N2 into the reaction mixture increased the isolated yield of 8 from 52 % to 82 % (Scheme 7). If the propynylated aromatics (13, 14)
Scheme 7

2227 The ADIMET Reaction: Synthesis and Properties of Poly(dialkylparaphenyleneethynylene)s
bear an alkyl group in a position ortho or meta to the propyne group, the yield (after crystallization and chromatography) of the dimers 18 or 19 exceeds 90 %, suggesting that their formation is quantitative.
Propynylnaphthalene 15 furnishes dinaphthylacetylene 20 in near quantitative yield. On the other hand, the Mori system performs somewhat less well if heteroatoms are present in the substrates, suggesting that the catalytic system is poisoned by the presence of the heteroatom through complexation and/or chelation of the active molybdenum center. Cyano groups and bromides/iodides inhibit the reactivity of the catalyst system. However, both propynylated phenols (16) and esters (17) give satisfactory dimerization results (21, 22).
Grubbs and Zuercher [15] have shown that alkene metathesis in the presence of alkynes leads to the transformation of the alkynes into alkenes in the final product. Thus, it was of importance to check if the reverse was true for alkyne metathesis utilizing the simple Mori system. Subjecting a series of propynylated styrenes and stilbenes 23–27 to the ‘‘shake-and- bake’’ catalysts led to the isolation of the dimers 28–32 in good to excellent yields after their precipitation from methanol (Scheme 8) [16]. It is noteworthy that unsupported double bonds are tolerated in the metathesis process forming divinyltolane 28 in good yields. Likewise, butadiene (25) and thiophene (26) containing substrates are dimerized, providing that the heteroatom or large p-system is far enough away from the reactive metathesis center.
Scheme 8