
Astruc D. - Modern arene chemistry (2002)(en)
.pdf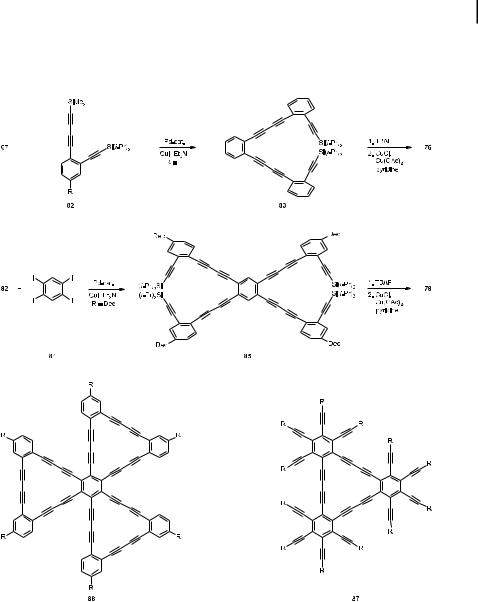
5.3 The Construction of Extended Aromatic Systems from Ethynyl Benzene Derivatives 183
diiodobenzene (67) in a modified Sonogashira coupling. The resulting 83 was subsequently deprotected by treatment with tetrabutylammonium fluoride (TBAF) and the hexayne thus formed was ring-closed by Eglinton coupling to give 76 (Scheme 20).
Scheme 20. The synthesis of several graphdiyne substructures.
Analogously, 82 (R ¼ Dec) was coupled with the tetraiodide 84 to give the polyacetylene 85, which, after desilylation, could be oxidized to the desired 78 [42]. The largest subsections of graphdiyne reported to date have been the hydrocarbons 86 (R ¼ tert-Bu and Dec) [43] and 87 (R ¼ tert-Bu) [44] (Scheme 20).
To study the aromatic character of dehydrobenzoannulenes by 1H NMR spectroscopy, it is desirable to replace one or more of the benzene rings in the above and related hydrocarbons

1845 From Acetylenes to Aromatics: Novel Routes -- Novel Products
by double bonds, and many of the respective model compounds have been prepared by coupling reactions similar to those presented so far [45]. Derivatives of several of the above hydrocarbons bearing polarizing functional groups have also been reported [46], as has the preparation of several derivatives in which the benzene ring has been replaced by a thiophene ring [47].
Compared to the purely organic dehydroannulenes, much less is known about systems that incorporate metal organic fragments. Nevertheless, due to the e orts of Bunz and coworkers, there has been steady progress in this area as well, and again interest in these compounds stems from their spectroscopic properties (especially their NMR behavior) and their potential uses in material science, one attractive goal being the preparation of metal-containing nanostructures. The preparation of the cyclobutadiene complex 91 is typical (Scheme 21).
Coupling of the cyclobutadiene complex 88 [48] with the protected acetylene 89 furnished the tetrayne 90, copper-mediated coupling of which, after removal of its acetylenic trimethylsilyl groups, yielded the organometallic complex 91 [49]. 1H NMR analysis of this
Scheme 21. The preparation of dehydrobenzannulenes containing metal organic fragments.

5.3 The Construction of Extended Aromatic Systems from Ethynyl Benzene Derivatives 185
and related dehydro[14]annulenes incorporating the bis(trimethylsilyl)cyclobutadiene-(cyclo- pentadienyl-cobalt) moiety suggest that the aromaticity of the fused cyclobutadiene complex might be stronger than that of benzene according to the ring-current criterion. The butterfly complex 92 [50] and the ferrocene derivative 93 [51] (Scheme 21) were synthesized by comparable coupling routes, starting from the building blocks 51 and 52, respectively. According to X-ray structural analysis, complexes such as 92 possess a concave structure and are of interest since the tetraethynylcyclobutadiene motif has been to suggested to play an important role in the formation of fullerenes such as C60 [52].
The central role of ortho-diethynylbenzene (47) as a construction unit in modern aromatic chemistry is also demonstrated by two other developments.
In 1982, Vollhardt and co-workers made a discovery that turned out to be of major importance with regard to the synthesis of numerous natural and non-natural products: addition of bis(trimethylsilyl)ethyne (94) to 47 in the presence of a Co catalyst led to the biphenylene derivative 95 in practically quantitative yield [53]. In fact, because of the steric bulk of the TMS substituents, self-trimerization of 94 does not take place, and it can be used as a solvent for the reaction. For the preparation of the [n]phenylenes, of which biphenylene is the smallest member, this conversion of acetylene units into aromatic rings remains the only general known protocol [54]. For example, when 95 was iododesilylated, the resulting diiodide 96 could be coupled with trimethylsilylethyne to give the bis(acetylene) 97, which, by repeating the addition step with 94 and removal of the protecting groups, was transformed into [3]phenylene (98, Scheme 22).
Scheme 22. A general route to linear [n]phenylenes.
When 47 was replaced by the tetraacetylene 99, the route to 98 could even be shortened: cycloaddition as described above provided the [3]phenylene derivative 100, which was desilylated to give 98 by treatment with potassium tert-butoxide in methanol [55].
The vast scope of this annelation sequence [54] has not only been demonstrated by the preparation of further linear [n]phenylenes such as 101, but also by the synthesis of angular representatives such as 102 and triangular systems such as 103 and 105 (Scheme 23) [56].
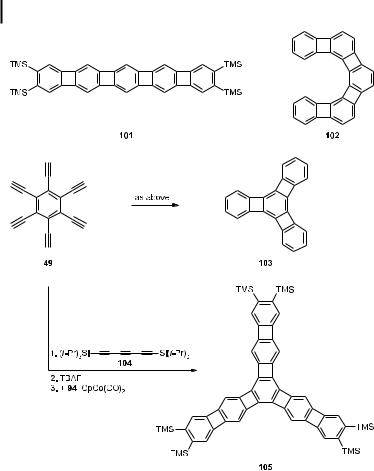
186 5 From Acetylenes to Aromatics: Novel Routes -- Novel Products
Scheme 23. Linear, angular, and triangular [n]phenylenes.
These latter aromatic compounds are of interest for the study of structural and bonding issues, since some of them show extreme bond alternation, suggesting the presence of discrete double and single bonds in aromatic rings [57].
In another recent development in aromatic synthesis, the Bergman cyclization [7] is beginning to be exploited for preparative purposes. This process has been studied for many years because of its importance with regard to the biological action of several marine antitumor antibiotics such as calicheamicin [58] and because of its interesting reaction mechanism [8]. Now, more and more synthetic applications are beginning to be reported in the literature [59].
A case in point, again involving a derivative of 47, is illustrated by the cycloaromatization of 106, a starting material that has two in-built radical traps. After the diradical 107 has been generated by the Bergman process, the 1,4-diyl is intercepted by the two a,b-unsaturated ester groups and the tetracyclic product 108 is formed (Scheme 24) [60].
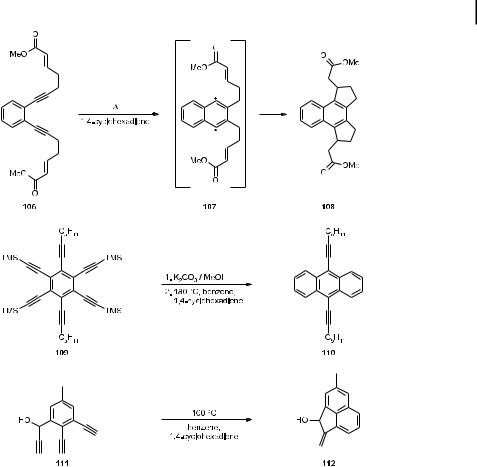
5.4 Bridged Aromatic Hydrocarbons Containing Triple Bonds (Cyclophynes) 187
Scheme 24. Using the Bergman cyclization for preparative purposes.
In another example, the hexaacetylene 109 – after deprotection with potassium carbonate in methanol – is subjected to typical Bergman trapping conditions, resulting in the formation of the anthracene derivative 110 [61]. As a third, more complex illustration, the aromatization of the triacetylene 111 may be considered. Here, the 1,4-diradical intermediate faces another triple bond as an internal trap, and, after hydrogen transfer from 1,4-cyclohexadiene, the tricyclic allylic alcohol 112 is produced [61].
5.4
Bridged Aromatic Hydrocarbons Containing Triple Bonds (Cyclophynes)
Ever since Cram started his systematic e orts to study bridged aromatic hydrocarbons and their derivatives half a century ago [62, 63], this field has had a strong influence on the development of aromatic chemistry [64–67]. In fact, after a period during which numerous cyclophane hydrocarbons were prepared and their chemical and structural properties were studied, their derivatives are now enjoying growing attention as chiral ligands in reagents

1885 From Acetylenes to Aromatics: Novel Routes -- Novel Products
used for asymmetric synthesis [68], as novel liquid crystalline materials [69], and as monomers in polymer chemistry [70], to name but a few recent applications. However, as in any active field, there is a strong need for the development of new synthetic methods providing these interesting aromatic compounds in ever increasing variety.
As found a few years ago [71], acetylenes that can be traced back to the diethynylbenzenes 45 and 47 are useful starting materials for the preparation of cyclophanes that possess triple bonds and triple-bond derived functionalities in their molecular bridges. Compared to cyclophanes bearing functional groups in benzene ring positions, relatively little is known about bridge-functionalized cyclophanes. That these – and especially acetylenic derivatives – have considerable potential in synthetic aromatic chemistry will be shown below.
Treating 113 (easily available from 45 by reaction of its dilithio derivative with formaldehyde and treatment of the resulting diol with phosphorus dibromide) with the so-called Mori reagent [72], a trimethylsilyl(tri-n-butyl)stannane/cesium fluoride combination that has been used very successfully in many debromination reactions [73], results in the production of the [6.6]paracyclophane 115 in acceptable yield. Since p-xylylene (p-quinodimethane) is known to dimerize to [2.2]paracyclophane, it may well be that the bis(cumulene) 114 is formed as an intermediate in this reaction. Since a stepwise process is also conceivable, further clarification of the mechanism is obviously desirable (Scheme 25).
Analogously, 116 and 117 have been prepared from the corresponding dibromides [74]. Applying the elimination protocol to the ortho-isomer of 113, the dibromide 118, results in
the formation of the ‘‘orthocyclophane’’ 120, i.e. in this case no dimerization of a reactive
Scheme 25. A new route to cyclophanes with bridges containing triple bonds.

5.4 Bridged Aromatic Hydrocarbons Containing Triple Bonds (Cyclophynes) 189
intermediate takes place. Nevertheless, 119 might have been formed initially, but since its two reactive [3]cumulene groups are in close proximity, intramolecular ring-closure to 120 is preferred over dimerization. At 77 %, the yield is again very good (Scheme 26) [74].
Scheme 26. Preparation and properties of the highly strained aromatic diyne 120.
Hydrocarbon 120 is a very reactive species since it can be reduced to the bis(olefin) 122 with lithium aluminum hydride in diethyl ether at room temperature, and trapped with tetracyclone (tetraphenylcyclopentadienone) to give the bis-adduct 121 in refluxing toluene. In both cases, the yields exceed 90 % [74].
When the carbocyclic aromatic core in the starting material is replaced by a heteroaromatic ring, systems such as 123 arise and Mori elimination/dimerization provides the dioxaand dithia[6.6]cyclophanes 125 (Scheme 27) [74].
The scope of this new approach to extended cyclophanes is further demonstrated by the conversion of the tetraethynyl derivatives 126 to the [10.10]cyclophane 128, the reactive [5]cumulene 127 formally being passed en route. The yields for the latter three reactions are low (between 20 and 30 %), but are still acceptable considering what has been accomplished [74].
Tetraacetylenes such as 115 and 116 contain the 1,5-hexadiyne group as a bridging element. Since the base-catalyzed isomerization of this unit to hexa-1,3-dien-5-yne (6) constitutes the basic reaction of Sondheimer’s annulene chemistry [75], it appeared attractive to attempt to apply this classic reaction of planar aromatic chemistry to a layered precursor and create three-dimensional relatives of Sondheimer’s dehydroannulenes. Indeed, both 115 and 116 could be isomerized to their fully conjugated isomers 129 and 130, respectively, by treatment with potassium tert-butoxide in tert-butanol, the original Sondheimer conditions (Scheme 28). From the X-ray structure obtained for 130, it was concluded that both hydro-
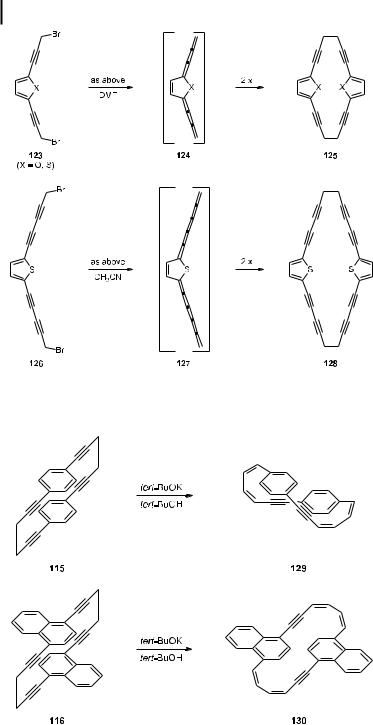
190 5 From Acetylenes to Aromatics: Novel Routes -- Novel Products
Scheme 27. Heterophanes with bridges containing triple bonds.
Scheme 28. The preparation of the three-dimensional annulenes 129 and 130.

5.4 Bridged Aromatic Hydrocarbons Containing Triple Bonds (Cyclophynes) 191
carbons 129 and 130 have a figure-8 structure (as indicated for 129), and are hence chiral [74].
Another type of chiral cyclophyne was obtained by applying the coupling methods developed by Haley to the chiral pseudo-ortho diacetylene 131 [76]. When this was connected to 2- (trimethylsilylethynyl)iodobenzene (132) the open tetraacetylene 133 resulted, which, after deprotection, was oxidatively closed to give 135. By a similar route, the non-benzo variant 134 was prepared, which could be characterized by X-ray structural analysis (Scheme 29) [76].
Scheme 29. Chiral paracyclophane/dehydrobenzannulene hybrids.
Hydrocarbons such as 134 and 135, so-called [2.2]paracyclophane/dehydrobenzoannulene hybrids (PC/DBAs), are not only of interest because of their stereochemical properties. Their electronic absorption spectra indicate that there must be electron delocalization through their open circuits. With their high degree of unsaturation, these layered hydrocarbons are also a step on the way to the triply-bridged cyclophane polyynes 136 and 138, generated in situ by Rubin [77] and by Tobe [78] by laser desorption time-of-flight mass spectrometry from appropriate precursor systems (these, in turn, produced by coupling reactions of various acetylenic building blocks). In the ICR mass spectrum (operating in the negative mode) of 136, partial dehydrogenation down to C60H16 was observed, suggesting the possibility of complete dehydrogenation to C60 (137). In the negative mode laser desorption mass spectrum of 138, the polyyne anion C60H6 was detected. Size-selective formation of C60þ and
was also observed, indicating a possible polyyne cyclization to the fullerene cage (Scheme 30).
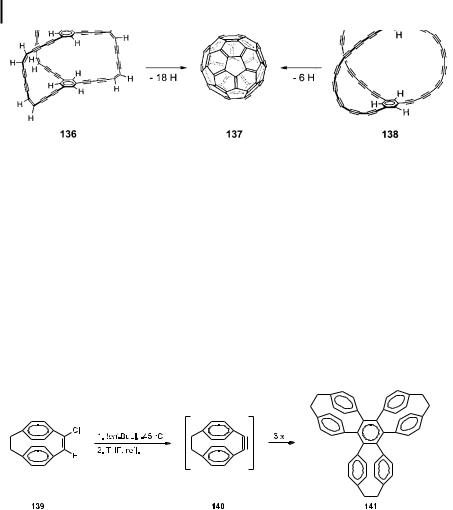
192 5 From Acetylenes to Aromatics: Novel Routes -- Novel Products
Scheme 30. Multibridged cyclophynes as precursors for C60 (137)?
Although representing rational syntheses of 137 – compared to the mode of formation of this parent fullerene from graphite [79] – it should be mentioned that these approaches do not provide isolable and spectroscopically charaterizable amounts of the precursor systems 136 and 138. Thus, it appears that for the time being the directed synthesis of these intermediates based on organochemical transformations is out of reach.
The first cyclophyne, incidentally, for which this name was also coined, was 1-cyclophyne (140), generated as a highly reactive intermediate from the vinyl chloride 139 by treatment with tert-butyllithium and heating of the resulting organolithio intermediate. Not surprisingly, 140 is unstable under the reaction conditions and trimerizes (inter alia) to trifoliaphane (141, Scheme 31) [80].
Scheme 31. A modern version of Berthelot’s benzene synthesis: trifoliaphane (141) from [2.2]paracyclophyne (140).
Although chemistry has clearly come a long way since the days of Berthelot – once again an acetylene has been converted into an aromatic ring.
Acknowledgement
I thank Cornelia Mlynek for drawing the reaction schemes.
References
1 M. Berthelot, Ann. Chem. Pharmacie |
in due course, became one of the |
1867, 141, 177–184. This journal later |
founding journals of the European Journal |
became Liebigs Annalen der Chemie, which, |
of Organic Chemistry. |