
Garrett R.H., Grisham C.M. - Biochemistry (1999)(2nd ed.)(en)
.pdf
5.3 ● The Many Biological Functions of Proteins |
121 |
Table 5.3
Biological Functions of Proteins and Some Representative Examples
Functional Class |
Examples |
|
|
Enzymes |
Ribonuclease |
|
Trypsin |
|
Phosphofructokinase |
|
Alcohol dehydrogenase |
|
Catalase |
|
“Malic” enzyme |
Regulatory proteins |
Insulin |
|
Somatotropin |
|
Thyrotropin |
|
lac repressor |
|
NF1 (nuclear factor 1) |
|
Catabolite activator protein (CAP) |
|
AP1 |
Transport proteins |
Hemoglobin |
|
Serum albumin |
|
Glucose transporter |
Storage proteins |
Ovalbumin |
|
Casein |
|
Zein |
|
Phaseolin |
|
Ferritin |
Contractile and motile proteins |
Actin |
|
Myosin |
|
Tubulin |
|
Dynein |
|
Kinesin |
Structural proteins |
-Keratin |
|
Collagen |
|
Elastin |
|
Fibroin |
|
Proteoglycans |
Scaffold proteins |
Grb 2 |
|
crk |
|
shc |
|
stat |
|
IRS-1 |
Protective and exploitive proteins |
Immunoglobulins |
|
Thrombin |
|
Fibrinogen |
|
Antifreeze proteins |
|
Snake and bee venom proteins |
|
Diphtheria toxin |
|
Ricin |
Exotic proteins |
Monellin |
|
Resilin |
|
Glue proteins |
|
|
122 Chapter 5 ● Proteins: Their Biological Functions and Primary Structure
Regulatory Proteins
A number of proteins do not perform any obvious chemical transformation but nevertheless can regulate the ability of other proteins to carry out their physiological functions. Such proteins are referred to as regulatory proteins. A well-known example is insulin, the hormone regulating glucose metabolism in animals. Insulin is a relatively small protein (5.7 kD) and consists of two polypeptide chains held together by disulfide cross-bridges. Other hormones that are also proteins include pituitary somatotropin (21 kD) and thyrotropin (28 kD), which stimulates the thyroid gland. Another group of regulatory proteins is involved in the regulation of gene expression. These proteins characteristically act by binding to DNA sequences that are adjacent to coding regions of genes, either activating or inhibiting the transcription of genetic information into RNA. Examples include repressors, which, because they block transcription, are considered negative control elements.
A prokaryotic representative is lac repressor (37 kD), which controls expression of the enzyme system responsible for the metabolism of lactose (milk sugar); a mammalian example is NF1 (nuclear factor 1, 60 kD), which inhibits transcription of the gene encoding the -globin polypeptide chain of hemoglobin. Positively acting control elements are also known. For example, the E. coli catabolite gene activator protein (CAP) (44 kD), under appropriate metabolic conditions, can bind to specific sites along the E. coli chromosome and increase the rate of transcription of adjacent genes. The mammalian AP1 is a heterodimeric transcription factor composed of one polypeptide from the Jun family of gene-regulatory proteins and one polypeptide from the Fos family of gene-regulatory proteins. AP1 activates expression of the -globin gene. These various DNA-binding regulatory proteins often possess characteristic structural features, such as helix-turn-helix, leucine zipper, and zinc finger motifs (see Chapter 31).
Transport Proteins
A third class of proteins is the transport proteins. These proteins function to transport specific substances from one place to another. One type of transport is exemplified by the transport of oxygen from the lungs to the tissues by hemoglobin (Figure 5.13a) or by the transport of fatty acids from adipose tissue to various organs by the blood protein serum albumin. A very different type is the transport of metabolites across permeability barriers such as cell membranes, as mediated by specific membrane proteins. These membrane transport proteins take up metabolite molecules on one side of a membrane, transport them across the membrane, and release them on the other side. Examples include the transport proteins responsible for the uptake of essential nutrients into the cell, such as glucose or amino acids (Figure 5.13b). All naturally occurring membrane transport proteins studied thus far form channels in the membrane through which the transported substances are passed.
Storage Proteins
Proteins whose biological function is to provide a reservoir of an essential nutrient are called storage proteins. Because proteins are amino acid polymers and because nitrogen is commonly a limiting nutrient for growth, organisms have exploited proteins as a means to provide sufficient nitrogen in times of need. For example, ovalbumin, the protein of egg white, provides the developing bird embryo with a source of nitrogen during its isolation within the egg. Casein is the most abundant protein of milk and thus the major nitrogen source for
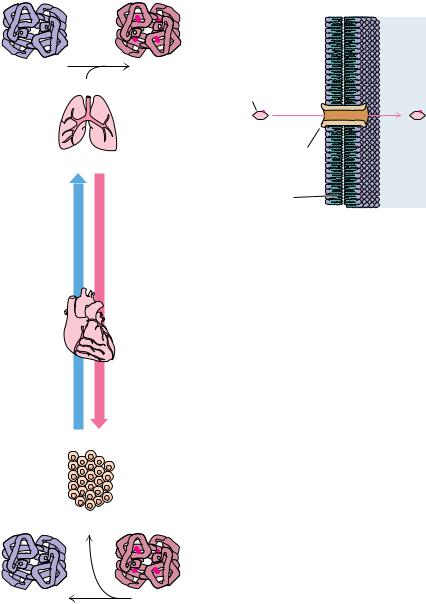
5.3 ● The Many Biological Functions of Proteins |
123 |
(a)
|
|
|
(b) |
|
|
|
|
Outside |
Inside |
Hemoglobin |
|
|
Hb(O2)4 |
|
(Hb) |
4 O2 |
|
|
|
|
Glucose |
|
||
|
|
|
|
|
Lungs |
|
|
|
|
|
|
|
Glucose transporter |
|
|
|
|
(a membrane protein) |
|
|
Venous |
Arterial |
Cell membrane |
|
|
|
|
||
|
rculationic |
circulation |
FIGURE 5.13 ● Two basic types of biological |
|
|
|
|
||
|
|
|
transport are (a) transport within or between |
|
|
|
|
different cells or tissues and (b) transport into |
|
|
|
|
or out of cells. Proteins function in both of |
|
Heart |
|
|
these phenomena. For example, the protein |
|
|
|
hemoglobin transports oxygen from the lungs |
||
|
|
|
to actively respiring tissues. Transport proteins |
|
|
|
|
of the other type are localized in cellular mem- |
|
|
|
|
branes, where they function in the uptake of |
|
|
|
|
specific nutrients, such as glucose (shown here) |
|
|
|
|
and amino acids, or the export of metabolites |
|
|
|
|
and waste products. |
|
Tissue
4 O2
Hemoglobin |
Hb(O2)4 |
(Hb) |
|
mammalian infants. The seeds of higher plants often contain as much as 60% storage protein to make the germinating seed nitrogen-sufficient during this crucial period of plant development. In corn (Zea mays or maize), a family of low molecular weight proteins in the kernel called zeins serve this purpose; peas (the seeds of Phaseolus vulgaris) contain a storage protein called phaseolin. The use of proteins as a reservoir of nitrogen is more efficient than storing an equivalent amount of amino acids. Not only is the osmotic pressure minimized, but the solvent capacity of the cell is taxed less in solvating one molecule of a polypeptide than in dissolving, for example, 100 molecules of free amino acids. Proteins can also serve to store nutrients other than the more obvious elements composing amino acids (N, C, H, O, and S). As an example, ferritin is a protein found in animal tissues that binds iron, retaining this essential metal so
124 Chapter 5 ● Proteins: Their Biological Functions and Primary Structure
that it is available for the synthesis of important iron-containing proteins such as hemoglobin. One molecule of ferritin (460 kD) binds as many as 4500 atoms of iron (35% by weight).
Contractile and Motile Proteins
Certain proteins endow cells with unique capabilities for movement. Cell division, muscle contraction, and cell motility represent some of the ways in which cells execute motion. The contractile and motile proteins underlying these motions share a common property: they are filamentous or polymerize to form filaments. Examples include actin and myosin, the filamentous proteins forming the contractile systems of cells, and tubulin, the major component of microtubules (the filaments involved in the mitotic spindle of cell division as well as in flagella and cilia). Another class of proteins involved in movement includes dynein and kinesin, so-called motor proteins that drive the movement of vesicles, granules, and organelles along microtubules serving as established cytoskeletal “tracks.”
Structural Proteins
An apparently passive but very important role of proteins is their function in creating and maintaining biological structures. Structural proteins provide strength and protection to cells and tissues. Monomeric units of structural proteins typically polymerize to generate long fibers (as in hair) or protective sheets of fibrous arrays, as in cowhide (leather). -Keratins are insoluble fibrous proteins making up hair, horns, and fingernails. Collagen, another insoluble fibrous protein, is found in bone, connective tissue, tendons, cartilage, and hide, where it forms inelastic fibrils of great strength. One-third of the total protein in a vertebrate animal is collagen. A structural protein having elastic properties is, appropriately, elastin, an important component of ligaments. Because of the way elastin monomers are cross-linked in forming polymers, elastin can stretch in two dimensions. Certain insects make a structurally useful protein, fibroin (a-keratin), the major constituent of cocoons (silk) and spider webs. An important protective barrier for animal cells is the extracellular matrix containing collagen and proteoglycans, covalent protein–polysaccharide complexes that cushion and lubricate.
Scaffold Proteins (Adapter Proteins)
Some proteins play a recently discovered role in the complex pathways of cellular response to hormones and growth factors. These proteins, the scaffold or adapter proteins, have a modular organization in which specific parts (modules) of the protein’s structure recognize and bind certain structural elements in other proteins through protein–protein interactions. For example, SH2 modules bind to proteins in which a tyrosine residue has become phosphorylated on its phenolic OOH, and SH3 modules bind to proteins having a characteristic grouping of proline residues. Others include PH modules, which bind to membranes, and PDZ-containing proteins, which bind specifically to the C-ter- minal amino acid of certain proteins. Because scaffold proteins typically possess several of these different kinds of modules, they can act as a scaffold onto which a set of different proteins is assembled into a multiprotein complex. Such assemblages are typically involved in coordinating and communicating the many intracellular responses to hormones or other signalling molecules (Figure 5.14). Anchoring (or targeting) proteins are proteins that bind other proteins, causing them to associate with other structures in the cell. A family of anchoring proteins, known as AKAP or A kinase anchoring proteins, exists in
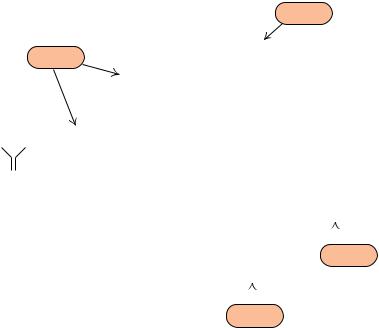

126 Chapter 5 ● Proteins: Their Biological Functions and Primary Structure
the hinges of insect wings. Certain marine organisms such as mussels secrete glue proteins, allowing them to attach firmly to hard surfaces. It is worth repeating that the great diversity of function in proteins, as reflected in this survey, is attained using just 20 amino acids.
5.4 ● Some Proteins Have Chemical Groups
Other Than Amino Acids
Many proteins consist of only amino acids and contain no other chemical groups. The enzyme ribonuclease and the contractile protein actin are two such examples. Such proteins are called simple proteins. However, many other proteins contain various chemical constituents as an integral part of their structure. These proteins are termed conjugated proteins (Table 5.4). If the nonprotein part is crucial to the protein’s function, it is referred to as a prosthetic group. If the nonprotein moiety is not covalently linked to the protein, it can usually be removed by denaturing the protein structure. However, if the conjugate is covalently joined to the protein, it may be necessary to carry out acid hydrolysis of the protein into its component amino acids in order to release it. Conjugated proteins are typically classified according to the chemical nature of their nonamino acid component; a representative selection of them is given here and in Table 5.4. (Note that comparisons of Tables 5.3 and 5.4 reveal two distinctly different ways of considering the nature of proteins—function versus chemistry.)
GLYCOPROTEINS. Glycoproteins are proteins that contain carbohydrate. Proteins destined for an extracellular location are characteristically glycoproteins. For example, fibronectin and proteoglycans are important components of the extracellular matrix that surrounds the cells of most tissues in animals. Immunoglobulin G molecules are the principal antibody species found circulating free in the blood plasma. Many membrane proteins are glycosylated on their extracellular segments.
LIPOPROTEINS. Blood plasma lipoproteins are prominent examples of the class of proteins conjugated with lipid. The plasma lipoproteins function primarily in the transport of lipids to sites of active membrane synthesis. Serum levels of low density lipoproteins (LDLs) are often used as a clinical index of susceptibility to vascular disease.
NUCLEOPROTEINS. Nucleoprotein conjugates have many roles in the storage and transmission of genetic information. Ribosomes are the sites of protein synthesis. Virus particles and even chromosomes are protein–nucleic acid complexes.
PHOSPHOPROTEINS. These proteins have phosphate groups esterified to the hydroxyls of serine, threonine, or tyrosine residues. Casein, the major protein of milk, contains many phosphates and serves to bring essential phosphorus to the growing infant. Many key steps in metabolism are regulated between states of activity or inactivity, depending on the presence or absence of phosphate groups on proteins, as we shall see in Chapter 15. Glycogen phosphorylase a is one well-studied example.
METALLOPROTEINS. Metalloproteins are either metal storage forms, as in the case of ferritin, or enzymes in which the metal atom participates in a catalytically important manner. We encounter many examples throughout this book of the vital metabolic functions served by metalloenzymes.
|
5.4 ● Some Proteins Have Chemical Groups Other Than Amino Acids |
127 |
|
Table 5.4 |
|
|
|
|
|
|
|
Representative Conjugated Proteins |
|
|
|
|
|
|
|
Class |
Prosthetic Group |
Percent by Weight |
|
|
|
(approx.) |
|
|
|
|
|
Glycoproteins contain carbohydrate |
|
|
|
Fibronectin |
|
|
|
-Globulin |
|
|
|
Proteoglycan |
|
|
|
Lipoproteins contain lipid |
|
|
|
Blood plasma lipoproteins: |
|
|
|
High density lipoprotein (HDL) ( -lipoprotein) |
Triacylglycerols, phospholipids, cholesterol |
75 |
|
Low density lipoprotein (LDL) ( -lipoprotein) |
Triacylglycerols, phospholipids, cholesterol |
67 |
|
Nucleoprotein complexes contain nucleic acid |
|
|
|
Ribosomes |
RNA |
60 |
|
Tobacco mosaic virus |
RNA |
5 |
|
Adenovirus |
DNA |
|
|
HIV-1 (AIDS virus) |
RNA |
|
|
Phosphoproteins contain phosphate |
|
|
|
Casein |
Phosphate groups |
|
|
Glycogen phosphorylase a |
Phosphate groups |
|
|
Metalloproteins contain metal atoms |
|
|
|
Ferritin |
Iron |
35 |
|
Alcohol dehydrogenase |
Zinc |
|
|
Cytochrome oxidase |
Copper and iron |
|
|
Nitrogenase |
Molybdenum and iron |
|
|
Pyruvate carboxylase |
Manganese |
|
|
Hemoproteins contain heme |
|
|
|
Hemoglobin |
|
|
|
Cytochrome c |
|
|
|
Catalase |
|
|
|
Nitrate reductase |
|
|
|
Ammonium oxidase |
|
|
|
Flavoproteins contain flavin |
|
|
|
Succinate dehydrogenase |
FAD |
|
|
NADH dehydrogenase |
FMN |
|
|
Dihydroorotate dehydrogenase |
FAD and FMN |
|
|
Sulfite reductase |
FAD and FMN |
|
|
|
|
|
|
HEMOPROTEINS. These proteins are actually a subclass of metalloproteins because their prosthetic group is heme, the name given to iron protoporphyrin IX (Figure 5.15). Because heme-containing proteins enjoy so many prominent biological functions, they are considered a class by themselves.
FLAVOPROTEINS. Flavin is an essential substance for the activity of a number of important oxidoreductases. We discuss the chemistry of flavin and its derivatives, FMN and FAD, in the chapter on electron transport and oxidative phosphorylation (Chapter 21).

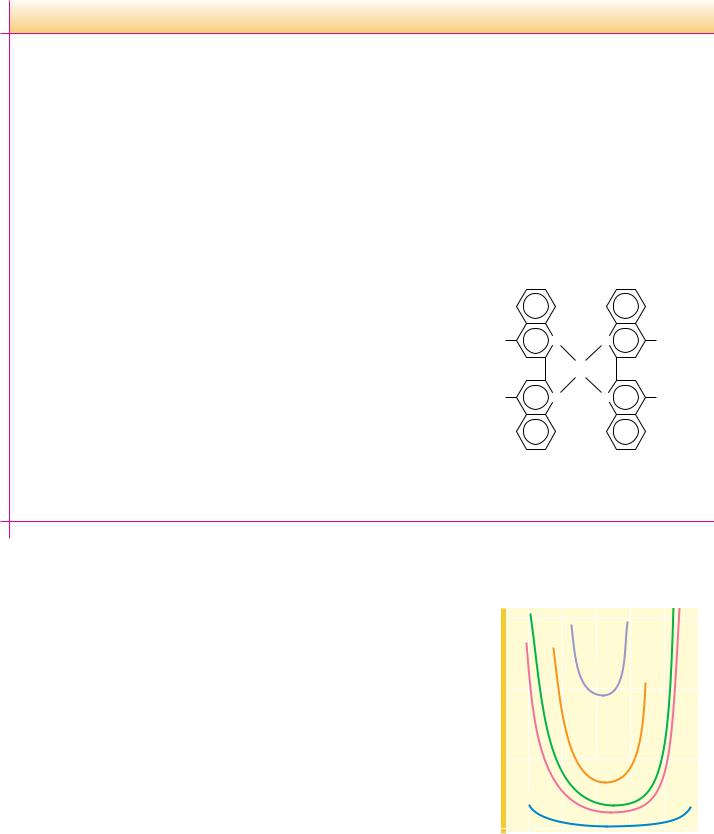
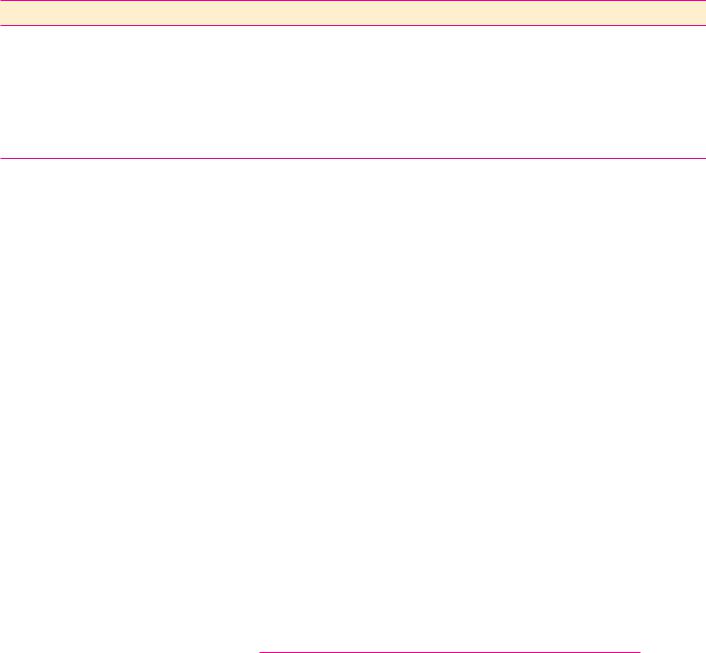
130 Chapter 5 ● Proteins: Their Biological Functions and Primary Structure
Table 5.5
Example of a Protein Purification Scheme: Purification of the Enzyme Xanthine Dehydrogenase from a Fungus
|
|
Volume |
Total |
Total |
Specific |
Percent |
Fraction |
(mL) |
Protein (mg) |
Activity* |
Activity† |
Recovery‡ |
|
|
|
|
|
|
|
|
1. |
Crude extract |
3,800 |
22,800 |
2,460 |
0.108 |
100 |
2. |
Salt precipitate |
,165 |
2,800 |
1,190 |
0.425 |
48 |
3. |
Ion exchange chromatography |
,65 |
,100 |
,720 |
7.2 |
29 |
4. |
Molecular sieve chromatography |
,40 |
,14.5 |
,555 |
38.3 |
23 |
5. |
Immunoaffinity chromatography§ |
,6 |
1.8 |
,275 |
152, |
11 |
*The relative enzymatic activity of each fraction in catalyzing the xanthine dehydrogenase reaction is cited as arbitrarily defined units.
†The specific activity is the total activity of the fraction divided by the total protein in the fraction. This value gives an indication of the increase in purity attained during the course of the purification as the samples become enriched for xanthine dehydrogenase protein.
‡The percent recovery of total activity is a measure of the yield of the desired product, xanthine dehydrogenase.
§The last step in the procedure is an affinity method in which antibodies specific for xanthine dehydrogenase are covalently coupled to a chromatography matrix and packed into a glass tube to make a chromatographic column through which fraction 4 is passed. The enzyme is bound by this immunoaffinity matrix while other proteins pass freely out. The enzyme is then recovered by passing a strong salt solution through the column, which dissociates the enzyme–antibody complex.
Adapted from Lyon, E. S., and Garrett, R. H., 1978. Journal of Biological Chemistry. 253:2604–2614.
it a partially hydrophobic character. Hydrophobic interaction chromatography is a protein purification technique that exploits this hydrophobicity (see Chapter Appendix).
A Typical Protein Purification Scheme
Most purification procedures for a particular protein are developed in an empirical manner, the overriding principle being purification of the protein to a homogeneous state with acceptable yield. Table 5.5 presents a summary of a purification scheme for a selected protein. Note that the specific activity of the protein (the enzyme xanthine dehydrogenase) in the immuno-affinity purified fraction (fraction 5) has been increased 152/0.108, or 1407 times the specific activity in the crude extract (fraction 1). Thus, xanthine dehydrogenase in fraction 5 versus fraction 1 is enriched more than 1400-fold by the purification procedure.
5.7 ● The Primary Structure of a Protein: Determining
the Amino Acid Sequence
In 1953, Frederick Sanger of Cambridge University in England reported the amino acid sequences of the two polypeptide chains composing the protein insulin (Figure 5.17). Not only was this a remarkable achievement in analytical chemistry but it helped to demystify speculation about the chemical nature of proteins. Sanger’s results clearly established that all of the molecules of a given protein have a fixed amino acid composition, a defined amino acid sequence, and therefore an invariant molecular weight. In short, proteins are well defined chemically. Today, the amino acid sequences of some 100,000 different proteins are known. Although many sequences have been determined from application of the principles first established by Sanger, most are now deduced from knowledge of the nucleotide sequence of the gene that encodes the protein.