
Physics of biomolecules and cells
.pdf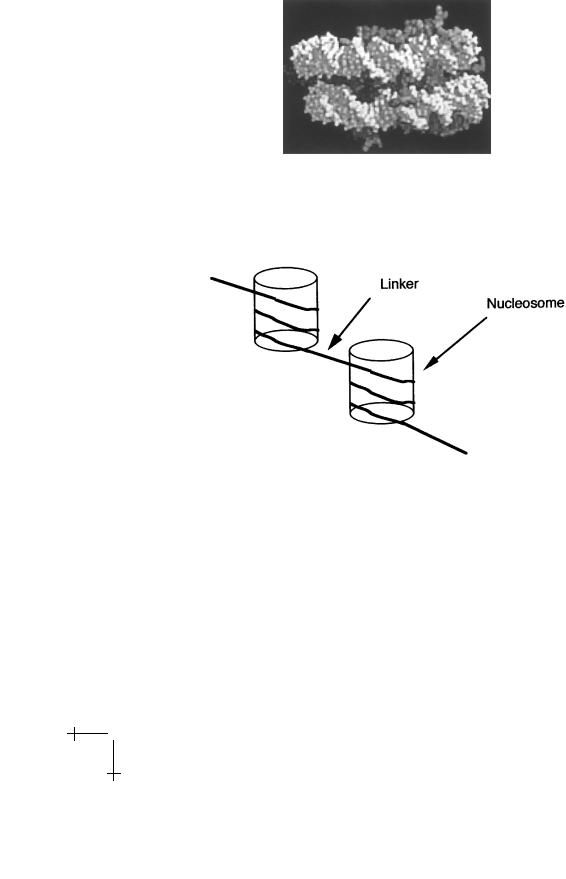
R.F. Bruinsma: Physics of Protein-DNA Interaction |
37 |
Fig. 16. Nucleosome structure (Ref. [29]).
a safety pin. As a consequence of the nucleosome structure, the 10 nm fiber has a spiral structure:
Fig. 17. The 10 nm fiber.
3.1.2 A mathematical experiment and White’s theorem
Figure 18 shows a mathematical experiment inspired by DNA condensation. Wrap a belt N spiral turns around the surface of a cylinder so that the belt is not twisted. If you slide a ruler along the belt, the ruler should not rotate. Now remove the cylinder and stretch the belt, without allowing the endpoints to rotate, for instance by first closing the belt into a loop using the buckle.
When the belt is straightened out, slide the ruler again along the belt. The ruler now makes N turns: we have transformed N turns in space (the
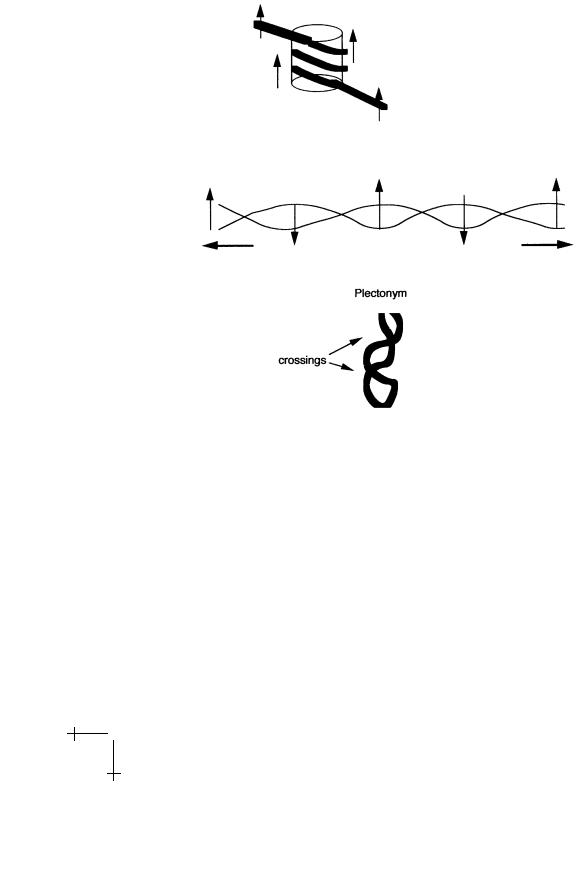
38 |
Physics of Bio-Molecules and Cells |
Fig. 18. A mathematical experiment. Spiral writhe is converted to twist, which in turn is converted to a plectonym.
spiral) into the N twist turns of the straight belt. We call the spatial turns “Writhe” and the turns of the straight belt “Twist”. Finally, bring the endpoints together, still not allowing end-point rotation. After the tension is removed, the belt is twisted up like a telephone wire into a shape known either as “interwound” or “plectonymic”. Using the ruler, verify that for large N the number of twists of the belt in the interwound state is much less than that for the straight belt. In this step, we reconverted twist into writhe, but the writhe is in a form di erent from the spiral we started out with. Note that you had to perform work to convert writhe into twist when you stretched the belt. This indicates that writhe is energetically less costly than twist.

R.F. Bruinsma: Physics of Protein-DNA Interaction |
39 |
The spiral (or “toroidal”) structure we started with is characteristic of DNA condensation in eukaryotes while the interwound state is characteristic of bacterial DNA folding: DNA extracted from bacteria has a branched plectonymic structure. Our mathematical experiment shows that there is no fundamental topological di erence between the two ways of folding DNA; they represent di erent forms of writhe and can be inter-converted.
Fig. 19. Sign convention for computing Wr.
The concept of writhe of an interwound curve can be quantified as follows. Move along the curve in a given direction. Assign each crossing of the curve with itself +1 or −1, according to the convention given in the picture. The writhe W r of the curve is the sum of these numbers over all the crossings. The twist T w is defined as the number of turns of the ruler in radians divided by 2π. If the mathematical experiment is repeated, you wil discover that – provided you do not open the buckle – the sum of T w
and W r is a constant, known as the Linking Number Lk: |
|
Lk = T w + W r. |
(3.1) |
This is known as White’s Theorem, and it can be demonstrated mathematically [30]. You can freely convert twist into writhe and vice versa but the sum remains fixed. If the belt is closed, then the linking number can be identified as the number of turns of the belt before you close it. You must be aware that the Lk = 0 state sometimes is defined with respect to the relaxed B DNA state and sometimes with respect to fully untwisted DNA with no double helix.
How do you compute the writhe if the belt is not a plectonym but, say, a spiral that twists in space without self-crossings? There exists a more precise definition of writhe. For a closed loop, W r is a double path integral along the contour C:
|
1 |
|
[dr |
dr2] |
|
r12 |
|
|
W r = |
|
C C |
1 |
× |
· |
|
· |
(3.2) |
4π |
|
|r12|3 |
|
|

40 |
Physics of Bio-Molecules and Cells |
If you compute this integral, you find that the writhe of a spiral with N spiral turns and a pitch angle α equals:
W r = ±N (1 + sin α) |
(3.3) |
with the + sign for a right handed spiral and the − sign for a left handed spiral (try to prove this yourself). What is the Lk? We already know that after stretching the belt you obtain N twisting turns. In this stretched state, there are no spatial turns so W r = 0. It then follows from White’s Theorem that the linking number must be +N or −N . (the convention is that right handed twist is positive). Applying again White’s Theorem, we find the twist:
T w = ±N sin α. |
(3.4) |
Let’s now apply White’s Theorem to the 10 nm fiber. Assume that the DNA of the 10 nm fiber is wrapped around the nucleosomes like a belt around a cylinder. In that case, we can simply adopt the preceding formulae. The Linking Number of the 10 nm fiber should then be approximately 1.75 times the number of nucleosomes N . When you measure Lk, you find that Lk is only about 1.0 times N . This is known as the “Linking Number Paradox”. It is not really a paradox: it only shows that that DNA is not wrapped around the nucleosome the way a belt is wrapped around a cylinder. White’s Theorem tells us that the nucleosome surface twists up the DNA to produce a negative contribution of about −0.75 per nucleosome. A mathematical theorem is providing us with detailed, microscopic information on the association of DNA with histones!
3.2 The worm-like chain
We now introduce the WLC model for DNA deformation [31]. It is based on the idea that the energy cost of small deformations that do not seriously alter the local DNA structures can be computed by elasticity theory. According to elasticity theory the energy cost of an infinitesimal deformation of a tube with uniform cross-section made of an isotropic elastic material is given by:
|
|
|
|
|
|
|
|
|
|
|
1 |
|
1 |
+ C |
dθ |
|
2 |
|
|
H = |
2 |
ds |
κ |
R2(s) |
ds |
· |
(3.5) |
The line integral runs along the center of the tube, with s the arc-length. In the first term, R(s) is the curvature radius of the center line with s the arclength along the center line (see Fig. 20, 1/R is called the curvature). If
ˆ |
2 |
ˆ |
2 |
t(s) is the tangent unit vector of the center line then (1/R(s)) |
|
= (dt/ds) . |
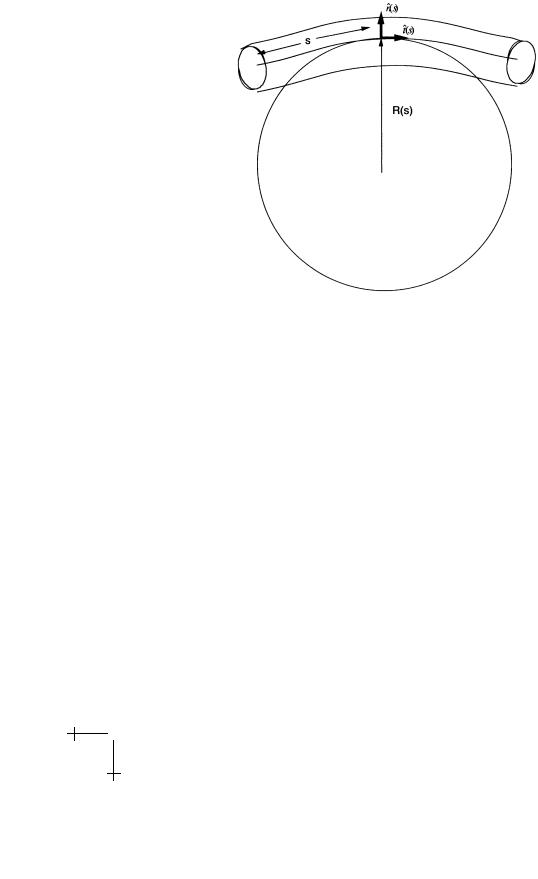
R.F. Bruinsma: Physics of Protein-DNA Interaction |
41 |
Fig. 20. The Worm-Like chain.
If this relation is used in the first term of equation (3.3) we obtain the wellknown one-dimensional non-linear sigma model. The material parameter
κ – with dimensions of Energy Length – is called the bending modulus (also denoted by A). The torque τ required to bend the tube along a circle of radius R is equal to κ/R.
The second term of equation (3.3) describes the twist energy. Let nˆ be a unit vector attached everywhere along the axis of the undeformed tube in a direction perpendicular to the tube axis. In the deformed state, dθ/ds is then the rate of rotation of nˆ(s) along s. (ˆn(s) is just the ruler of our mathematical experiment). Note that the twist modulus C has the same dimensions as the bend modulus.
DNA is of course not well described as being composed of an isotropic elastic material so why does equation (3.5) make any sense? That is because the mathematical form for H can be valid even if elasticity theory does not apply. Assume that if DNA is only very weakly deformed, the energy cost must be an integral along s of some function of the bend, twist, and torsion. If you assume that this expression is an analytic function of bend, twist, and torsion and if you expand this function in a Taylor series to lowest order, you again obtain equation (3.5) if you demand that DNA has no intrinsic bend, twist, or torsion.

42 |
Physics of Bio-Molecules and Cells |
3.2.1 Circular DNA and the persistence length
As a first application of the WLC, consider a closed strand of DNA that lies along a circle of radius R (no twisting). The elastic energy cost (Eq. (3.5)) equals
H = 2πκ/R. |
(3.6) |
For increasing R, H becomes smaller than the thermal energy kBT for R ξ with ξ = kBT /κ known as the “persistence length”. If R ξ, then thermal fluctuations will deform the large circle by introducing smaller loops and turns with a characteristic size equal to ξ. On the other hand, if R ξ, then it is not possible for thermal fluctuations to deform the circle. We conclude that thermal fluctuations are not able to deform DNA on length-scales less than ξ. It is possible to microscopically observe the thermal fluctuations – or Brownian Motion – of DNA strands by attaching fluorescent markers and measure the persistence length ξ (about 500 ˚A). As an application, we can calculate the bending energy cost of the lac repressor loop. The distance between the two lac repressor operator sequences is about 300 ˚A. Assuming that the DNA spans a 300 ˚A perimeter circle between the operator sequences and using equation (3.6) gives a bending energy H for the loop of more than 30 kBT . This is an overestimate, but the elastic energy cost of the loop is indeed substantial compared with the typical protein-DNA binding energy.
By the way, the precise definition of the persistence length is as a cor-
relation length for orientational fluctuations: |
|
|
|
|||||||
lim |
|
ˆ |
|
ˆ |
|
|
exp( |
|
s/ξ) |
(3.7) |
|
t(0) |
· |
t(s) |
|
|
− |
||||
s→∞ |
|
|
|
|
|
where denotes a thermal average and ˆ( ) is again the tangent unit
. . . t s
vector.
3.2.2 Nucleosomes and the Marky–Manning transition
We now apply the WLC to the nucleosome. Let L be the length of DNA wrapped around the core, with L less than or equal to 140 bp. Treat the core as a cylinder of radius R equal to 5 nm. The bending energy cost is then H = 12 κL/R2 according to equation (3.5) (there is some twist energy too but we will neglect it). Assuming κ/kBT = 500 ˚A, one finds that wrapping the full 140 bp around the core requires about 36 kBT in energy per nucleosome. The total human genome is about 109 bp, requiring about 109/200 nucleosomes. The total amount of DNA elastic energy stored in the nucleus is about 108 kBT . That is serious energy. In the figure below [32], you see what some of this energy does when you decondense the chromatin by chemical treatment: it causes a DNA explosion!

R.F. Bruinsma: Physics of Protein-DNA Interaction |
43 |
Fig. 21. Decondensed Chromatin (from Ref. [32]).
This bending energy cost must be compensated by some form of attractive interaction between the core and the DNA. This attraction is due to electrostsatics and will be discussed further in Section 4. Here we only need the fact that the binding between DNA and histones is nearly sequenceinsensitive so the binding energy should mainly depend on how much DNA is associated with the nucleosome, i.e., on L. We thus introduce an (unknown) adhesion energy λ per unit length, with λ to be determined experimentally. Adding bending and adhesion energies gives:
E(L) = |
1 |
κ/R2 − λ L. |
(3.8) |
2 |
There are now two possibilities: if 1/2κ/R2 does not exceed λ, then DNA will wrap around the core to the maximum amount since E(L) decreases with increasing L. If 1/2κ/R2 exceeds λ, then E(L) is minimized by L = 0 so there is no complexation. This “unwrapping” transition of Marky and Manning [33] was studied experimentally by Yaeger and von Holde [34] (see
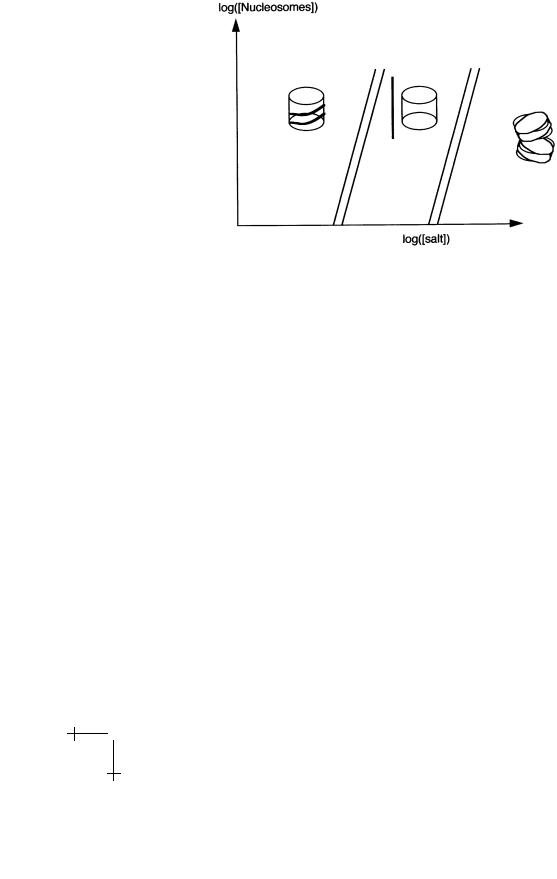
44 |
Physics of Bio-Molecules and Cells |
Fig. 22. Phase Diagram of a Nucleosome Solution.
Fig. 22). They changed the salt concentration in a dilute solution of nucleosome particles – in order to change λ – and found a first-order unwrapping transition for salt concentrations somewhat above the physiological level of 0.1 M. At even higher salt concentrations, the core fell apart. That indicates that nucleosome particles are rather close to the unbinding transition under physiological conditions. Since we know the bending modulus of DNA, we can estimate this way that λ is of order κ/R2, which is about 0.2 kBT /A, or about 0.6 kBT /A per bp.
It is actually a very reasonable design strategy for nucleosomes to be close to the unwrapping transition. Chromatin decondensation can then take place by minor chemical changes of the nucleosome core (e.g., by reducing the positive charge of the histones through acetylation).
Polach and Widom developed a clever way of measuring λ in an independent way [35]. Recall that digestion enzymes can cut DNA strands that are not protected by proteins. If the nucleosome “operating point” indeed is close to the Marky–Manning transition, we might expect significant thermal fluctuations in the wrapping length L. This can be checked by allowing the nucleosome solution to be incubated for a while with digestion enzymes. According to the Boltzmann Distribution, the probability distribution P (L) of the wrapping lengths should be an exponential function of

R.F. Bruinsma: Physics of Protein-DNA Interaction |
45 |
|||
L (using Eq. (3.6)): |
|
|
|
|
P (L) exp |
1 |
κ/R2 − λ (Lmax − L)/κBT |
|
(3.9) |
|
||||
2 |
with Lmax the maximum wrapping length. The distribution of wrapping lengths was indeed found to be exponential, which allowed a separate measurement of λ (obtaining a value of the same order as our crude estimate).
The fact that there are significant fluctuations in the DNA length is interesting by itself for a number of reasons. The possibility of formation by thermal fluctuations of small, open DNA loops along the wrapped length provides a mechanism for the mobility of nucleosomes along DNA without the nucleosome becoming detached from the DNA [36], which indeed has been observed experimentally.
3.2.3 Protein-DNA interaction under tension
Our next example of the WLC concerns what happens with DNA associated proteins when the DNA is placed under tension. We saw earlier that the binding of many proteins to DNA produces a bend or a kink in the DNA. Tension will increase the energy cost of producing the kink. Let’s compute this energy cost? Assume that the protein binds at the midpoint of a strand of length L. Let the kink angle be π − 2α (see figure). Now place the DNA strand under a tension F .
Fig. 23. A protein/DNA complex under tension.
Let s be the arc-length along the DNA, with s = 0 the kink location. The curvature 1/R(s) at s is then dθ/ds, with θ the angle between the tangent and the direction along which the tension is applied. The boundary conditions on θ(s) for large L are θ(0) = α and θ(∞) = 0. The total energy is the WLC bending energy plus the work −F Xp performed by the DNA against the tension, with Xp the length of the projection of the DNA along

46 |
Physics of Bio-Molecules and Cells |
|
|||||||||
the coordinate axis: |
|
|
|
|
|
|
|
|
|
|
|
|
|
|
|
|
|
|
dθ |
|
|||
|
|
|
L/2 |
1 |
|
|
2 |
|
|||
|
E = 2 |
|
|
ds |
|
κ |
|
|
− F cos θ · |
(3.10) |
|
|
0 |
|
2 |
ds |
Equation (3.10) is a famous 19’th century problem of elasticity known as the “Elastica”. The Euler–Lagrange equation δE/δθ = 0 minimizing E reads:
d2θ |
− F sin θ = 0. |
(3.11) |
κ ds2 |
This happens to be the equation of motion for an ideal pendulum of length l, mass M if we make the following identifications:
κ → Ml
F → Mg s → time
and θ(s) the angle between the pendulum and the upwards vertical direction (so θ = π is the equilibrium position). The particular pendulum solution we are interested in starts in an upside-down position and then rotates clockwise until the pendulum makes an angle α with the vertical:
Fig. 24. The Pendulum Analog.
This must be matched with a similar solution that makes a counterclockwise turn. Although the general solution can be expressed in terms of ellipitic integrals, we will restrict ourselves to the case that α 1. In that case, we can linearize the Euler–Lagrange equation:
d2θ |
− F θ = 0. |
(3.12) |
κ ds2 |
Note that this is not the harmonic oscillator equation, and the solution is an exponential, not a trigonometric function:
θ(s) = |
±α exp(−|s|/λ) |
|
||||
λ = |
|
|
κ |
· |
(3.13) |
|
|
|
|
F