
Matta, Boyd. The quantum theory of atoms in molecules
.pdf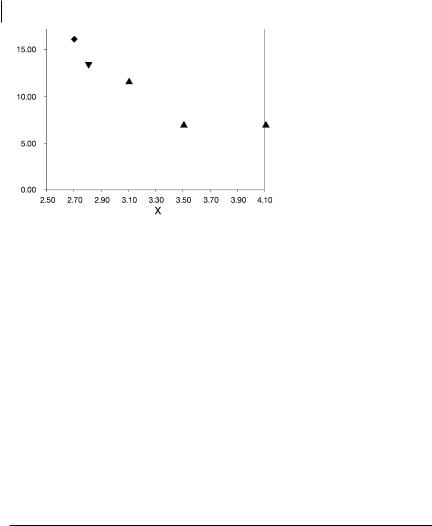
152 6 The ELF Topological Analysis Contribution to Conceptual Chemistry and Phenomenological Models
Fig. 6.8 Dependence of V(C, X) volume (bohr3) on Dw. Csecond period elements, Dthird period elements, Yfourth period elements.
with increasing electronegativity is a consequence of the charge-shift nature of the bonds [73, 116].
To check the VSEPR assumptions we consider:
1.the V(X), VðX; HÞ, and VðC; XÞ basins of CH3XHn (X ¼ F, Cl, Br, O, N; n ¼ 0; 1; 2); and
2.the V(X) and VðX; HÞ basins of H2O, H2S, H2Se, NH3, PH3 and AsH3.
The volumes of these basins are reported in Table 6.2. Around the X center in the CH3XHn series the VðC; XÞ basins are always smaller than the V(X) basins, in
Table 6.2 V(X), V(X, H), and V(C, X) basin volumes (a.u.).
Molecule |
V(X) |
V(X, H) |
V(C, X) |
|
|
|
|
NH3 |
108.9 |
109.0 |
|
PH3 |
287.4 |
178.0 |
|
AsH3 |
297.4 |
183.8 |
|
H2O |
75.6 |
86.0 |
|
H2S |
228.2 |
139.5 |
|
H2Se |
231.3 |
146.0 |
|
CH3NH2 |
96.8 |
103.5 |
11.6 |
CH3OH |
69.1 |
80.6 |
7.0 |
CH3F |
48.5 |
|
7.0 |
CH3Cl |
152.1 |
|
13.3 |
CH3Br |
168.6 |
|
16.1 |
|
|
|
|

6.5 Examples of the Correspondence Between ELF Basins and the Domains of the VSEPR Model 153
agreement with assumption 1, that lone-pair basins are always larger than the bonding domains on the same center. They are also in agreement with assumption 2, that the size of a bonding domain decreases when the electronegativity of the ligand increases. For the period-2 molecules NH3, H2O, CH3NH2, and CH3OH, however, the V(X) basins are almost equal to, or slightly smaller than the V(X) basins, which is not in agreement with assumption 2.
This is also true for the third and fourth period hydrides but not for the period- 2 hydrides, for which the V(X) basins are almost equal to, or slightly smaller than, the ðX; HÞ basins, which is not in agreement with assumption 1. The ELF analysis therefore supports the first VSEPR assumption, i.e. that nonbonding or lone-pair domains are larger than bonding domains in the valence shell of the same central atom, but it is not in accord with the second assumption for hydrogen ligands. Gillespie and Robinson [117] have, however, recently concluded from a survey of bond angles and their relationship with ligand radii that the most important factor determining bond angles are ligand sizes rather than the size of bonding pair domains. They have shown, however, that the VSEPR and ligand–ligand repulsion models usually lead to the same conclusions, except for hydrogen ligands the particularly small size of which is the key factor in determining geometry. For a given ligand and central atoms of the same group, the volume of the VðA; XÞ basin increases with period number and, to some extent, with decreasing electronegativity. For example, the VðSi; FÞ basin volume is twice that of VðC; FÞ.
6.5
Examples of the Correspondence Between ELF Basins and the Domains of the VSEPR Model
6.5.1
Octet Molecules
6.5.1.1 Hydrides (CH4, NH3, H2O)
In these molecules ELF shows:
1.a basin corresponding to the central atom core; and
2.four, three, and two disynaptic basins, respectively, corresponding to the bonding domains of the VSEPR model, and one or two monosynaptic basins corresponding to the lone-pair domains of the VSEPR model.
Hydrides are unique in that hydrogen has no core. Accordingly there is no core basin. These basins could also be considered to correspond to protonated lone pairs, i.e. as protonated monosynaptic basins. As shown in Table 6.3, the populations of the AaH bonds follow the expected trend – they decrease as the electronegativity of the central atom increases, that is, when bond polarity increases.
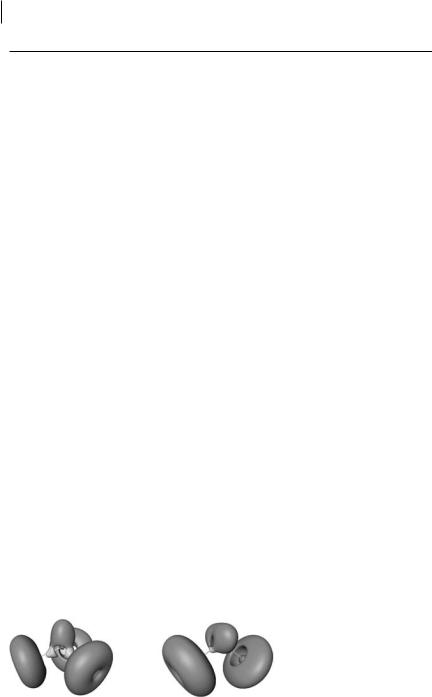
1546 The ELF Topological Analysis Contribution to Conceptual Chemistry and Phenomenological Models
Table 6.3 V(A), V(A, X), and V(X) basin populations (e), (A ¼ C, N, O, Si; X ¼ H, F, Cl).
Molecule |
V(A) |
(A, X) |
V(X) |
|
|
|
|
CH4 |
|
1.98 |
|
NH3 |
2.16 |
1.91 |
|
H2O |
2.27 |
1.66 |
|
CF4 |
|
1.31 |
6.54[a] |
SiCl4 |
|
1.61 |
6.40[a] |
|
|
|
|
a The F and Cl lone pairs are merged in a single basin.
6.5.1.2AX4 (CH4, CF4, SiCl4)
For these molecules ELF reveals a basin corresponding to the core, four disynaptic basins corresponding to the four bonding basins of the VSEPR model, and a monosynaptic basin on each ligand corresponding to the sum of the lone-pair domains of the VSEPR model. Because of the necessary cylindrical symmetry around a single bond the nonbonding electrons do not, in fact, form three nonbonding pairs but have a most probable location in a ring perpendicular to the bond axis and the basin has a corresponding toroidal shape. In CF4 and SiCl4 the population of each of the disynaptic basins is less than two electrons whereas the populations of the monosynaptic basins are correspondingly larger (Table 6.3). These populations are characteristic of polar bonds, the extent to which the population of the disynaptic basins is less than two electrons is a measure of their bond polarity.
6.5.1.3AX3E and AX2E2 (NCl3, OCl2)
The ELF localization domains of these molecules are displayed in Fig. 6.9. They look similar to the corresponding hydrides except that the ligands also have a monosynaptic basin as in the AX4 molecules. It can happen that the expected disynaptic basin does not exist, presumably because of the large electronegativity di erence. The monosynaptic (lone-pair) basins seem larger than the bonding basins, and this is verified by their populations, consistent with the VSEPR model
Fig. 6.9 ELF ¼ 0.75 localization domains of NCl3 (left) and OCl2 (right).

6.5 Examples of the Correspondence Between ELF Basins and the Domains of the VSEPR Model 155
Fig. 6.10 ELF ¼ 0.75 localization domains of PCl5 (left) and SF6 (right).
that postulated that the lone-pair domains take up more space around the core than the bonding domains.
6.5.2
Hypervalent Molecules
6.5.2.1PCl5 and SF6
Hypervalent molecules are those in which the octet rule is violated because their Lewis structures have more than eight electrons in the valence shell of the central atom: PCl5 and SF6 are typical examples. ELF plots for these molecules are shown in Fig. 6.10. In each example there are core basins for each atom, disynaptic basins corresponding to the domains of the VSEPR model, and a monosynaptic basin on each ligand. The disynaptic basins have a population of less than two electrons, showing that the bonds are polar.
6.5.2.2SF4 and ClF3
According to the VSEPR model these molecules have, respectively, one and two lone-pair domains in the valence shell of the central atom. The ELF plots in Fig. 6.11 show the monosynaptic basins corresponding to these lone-pair domains.
6.5.2.3AX7 and AX6E Molecules
The structures of molecules with seven ligands cannot be predicted with certainty by the VSEPR model. The points-on-a-sphere model shows that the three most probable structures are the pentagonal pyramid, the mono-capped distorted octa-
Fig. 6.11 ELF ¼ 0.75 localization domains of SF4 (left) and ClF3 (right).
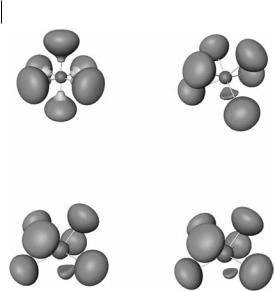
156 6 The ELF Topological Analysis Contribution to Conceptual Chemistry and Phenomenological Models
Fig. 6.12 ELF ¼ 0.75 localization domains of BrF6 (top left), SeF6 2 (top right), IF6 (bottom left), and XeF6 (bottom right).
hedron, and the mono-capped trigonal prism. All known seven-coordinated molecules, for example IF7 and TeF7 , have a pentagonal bipyramid structure. It might be expected, therefore, that AX6E molecules, for example TeCl6 2 , BrF6 , SeF6 2 , and XeF6, would have a structure a based on the pentagonal bipyramid with a lone pair in an axial position (the least crowded position), that is to say, a pentagonal pyramid. No AX6E molecules have this shape, however; they are either octahedral (Oh, for example TeCl6 2 and BrF6 , or a have a C3v distorted octahedral shape, for example SeF6 2 and XeF6. It has been proposed that in the octahedral molecules the ligands take up all the space in the valence shell leaving no room for nonbonding electrons, which form an outer shell of the core. In C3v molecules the crowding of the ligands is not as great, so there is room for some nonbonding density in the valence shell forming what has been described as a ‘‘weak lone pair’’. The ELF plot for these molecules is in good agreement with this proposal. In Fig. 6.12 it can be seen there is a nonbonding basin in the valence shell of the central atom corresponding to the proposed weak lone pair. This basin is also involved in strong exchange with the core, consistent with the idea that the weak lone pair has partial lone-pair and partial core character.
6.5.3
Multiple Bonds
6.5.3.1C2H4 and C2H2
ELF plots for these two molecules are given in Fig. 6.13. In C2H4 there are four disynaptic basins corresponding to the four CaH bonds, and a large disynaptic
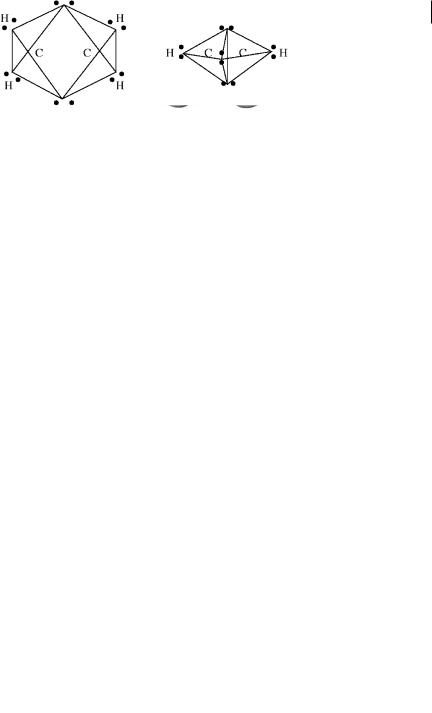
6.5 Examples of the Correspondence Between ELF Basins and the Domains of the VSEPR Model 157
Fig. 6.13 ELF ¼ 0.75 localization domains of C2H2 (left) and C2H4 (right).
basin corresponding to the CbC double bond. This basin has two maxima, one on each side of the CC axis, and splits into two basins at a su ciently high value. These two basins are consistent with the VSEPR model of the double bond which consists of two bent bonds.
In the ELF plot for C2H2 there are two disynaptic basins corresponding to the two CH bond domains and a toroidal basin that surrounds the CC axis. This basin corresponds to the six bonding electrons. In the VSEPR model there would be three bonding pairs with their most probable locations located around the CC axis. As Linnett pointed out many years ago in his double quartet theory, however, there is no reason for the six bonding electrons to be localized as pairs, or indeed for any electrons that are not on the bond axis of a linear molecule to be fixed in localized pairs. Linnett proposed that the six bonding electrons have their most probable positions on a circle perpendicular to the bond axis with opposite spins alternating (Fig. 6.14). The observed toroidal basin in ELF corresponds to the Linnett model.
6.5.3.2Si2Me4 and Si2Me2
According to the Lewis model these molecules have double and triple bonds just as in ethene and ethyne. There has, however, been much discussion about the real nature of these bonds, because their properties are not in agreement with these Lewis structures. In particular the molecules are not linear but have a trans bent shape. The ELF basins, however, give a clear picture of the electron distribution in these molecules, in which it can be seen that the formally multiple bond-
Fig. 6.14 Linnett representations of C2H4 (left) and C2H2 (right).
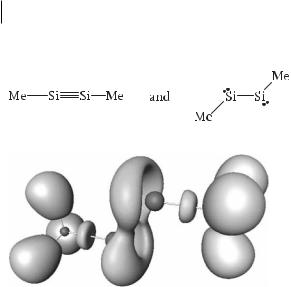
158 6 The ELF Topological Analysis Contribution to Conceptual Chemistry and Phenomenological Models
ing electrons also have some nonbonding or lone-pair character. The molecule Me2Si2 (Fig. 6.15) can, for example, be described as a resonance hybrid of:
Fig. 6.15 ELF ¼ 0.75 localization domains of Si2Me2.
This structure is consistent with the suggestion that only the most electronegative elements can hold two or three mutually repelling electron pairs close together in a multiple bond – these are principally the second period elements, C, N, and O, which do, indeed, form the vast majority of known multiple bonds.
6.6 Conclusions
ELF topological analysis provides a mathematical bridge between quantum mechanics and chemistry which relies on the one hand on statistical interpretation and on the other hand on the theory of dynamical systems. This approach shares the dynamical system theory, a common mathematical method of partitioning, with the quantum theory of atoms in molecules. The di erence is in the nature of the potential function used and, therefore, the nature of the properties investigated. QTAIM is rightly claimed to be rooted in physics rather than in chemistry and the objective of its partition scheme is, accordingly, to define open quantum systems within which the virial theorem is valid. QTAIM does not, moreover, attempt to define the valence shell of an atom in a molecule. The goal of ELF partitioning is clearly to define basins of attractor corresponding to chemical concepts such as core and valence shells, bonds, and lone pairs. This is achieved at the expense of the physical meaning of the basins, which is lost. Another advantage of the ELF approach is that it provides pictures of the bonding in molecules which display pictorially the electron domains of the VSEPR model [13] and, in particular, provide evidence of the lone pairs [118]. ELF analysis has helped to refine

References 159
the conclusions of the VSEPR model, in particular for so-called hypervalent molecules [56], and to extend its applicability to the d0 molecules of period 4 metals by considering basins of the core external shell [66]. Analysis of the basin volumes shows that the volumes of the core basins and of the structural features of their outermost shell are not always negligible. This analysis also enables rationalization of the subtle e ects of electronegativity and, therefore, is a means of improving the VSEPR model.
References
1 |
R. Thom, Paraboles et Catastrophes, |
14 |
R. J. Gillespie, Coord. Chem. Chem. |
|
Flammarion, Paris, 1983. |
|
Rev. 2000, 197, 51–69. |
2 |
R. Daudel, Les Fondements de la |
15 |
R. J. Gillespie, E. A. Robinson, Chem. |
|
Chimie The´orique, Gauthier–Villars, |
|
Soc. Rev. 2005, 34, 396–407. |
|
Paris, 1957. |
16 |
B. Silvi, A. Savin, Nature 1994, 371, |
3 |
R. Daudel, in Aspects de la Chimie |
|
683–686. |
|
quantique Contemporaine (Eds. R. |
17 |
U. Ha¨ussermann, S. Wengert, R. |
|
Daudel, A. Pullman), Editions du |
|
Nesper, Angew. Chem. Int. Ed. Engl. |
|
Center National de la Recherche |
|
1994, 33, 2069–2072. |
|
Scientifique, Paris, 1971 p. 70. |
18 |
A. D. Becke, K. E. Edgecombe, |
4 |
C. Aslangul, R. Constanciel, R. |
|
J. Chem. Phys. 1990, 92, 5397–5403. |
|
Daudel, P. Kottis, in Advances in |
19 |
N. O. J. Malcolm, P. L. A. Popelier, |
|
Quantum Chemistry (Ed. P.-O. |
|
Faraday Discuss. 2003, 124, 353–363. |
|
Lo¨wdin), Academic Press, New York, |
20 |
G. N. Lewis, J. Am. Chem. Soc. 1916, |
|
vol. 6, 1972 pp. 93–141. |
|
38, 762–786. |
5 |
C. E. Shannon, W. Weaver, The |
21 |
G. N. Lewis, Valence and the Structure |
|
Mathematical Theory of Communi- |
|
of Atoms and Molecules, Dover, New |
|
cation, The University of Illinois |
|
York, 1966. |
|
Press, Urbana, 1949. |
22 |
B. Silvi, Phys. Chem. Chem. Phys. |
6 |
R. H. Abraham, C. D. Shaw, |
|
2004, 6, 256–260. |
|
Dynamics: The Geometry of Behavior, |
23 |
B. Silvi, J. Phys. Chem. A 2003, 107, |
|
Addison Wesley, 1992. |
|
3081–3085. |
7 |
R. H. Abraham, J. E. Marsden, |
24 |
J. F. Dobson, J. Chem. Phys. 1991, 94, |
|
Foundations of Mechanics, Addison |
|
4328–4333. |
|
Wesley, 1994. |
25 |
A. Savin, O. Jepsen, J. Flad, O. K. |
8 |
R. F. W. Bader, Atoms in Molecules: A |
|
Andersen, H. Preuss, H. G. von |
|
Quantum Theory, Oxford Univ. Press, |
|
Schnering, Angew. Chem. Int. Ed. |
|
Oxford, 1990. |
|
Engl. 1992, 31, 187–190. |
9 |
R. J. Gillespie, R. S. Nyholm, Quart. |
26 |
J. K. Burdett, T. A. McCormick, |
|
Rev. Chem. Soc. 1957, 11, 339–380. |
|
J. Phys. Chem. A 1998, 102, |
10 |
R. J. Gillespie, Molecular Geometry, |
|
6366–6372. |
|
Van Nostrand Reinhold, London, |
27 |
R. Nalewajski, A. Koster, S. Escalante, |
|
1972. |
|
J. Phys. Chem. A 2005, 109, |
11 |
R. J. Gillespie, I. Hargittai, The |
|
10038–10043. |
|
VSEPR Model of Molecular Geometry, |
28 |
A. Burkhardt, U. Wedig, H. G. von |
|
Allyn and Bacon, Boston, 1991. |
|
Schnering, A. Savin, Z. anorg. allgem. |
12 |
R. J. Gillespie, Chem. Soc. Rev. 1991, |
|
Chem. 1993, 619, 437–441. |
|
21, 59–69. |
29 |
A. Savin, B. Silvi, F. Colonna, Can. J. |
13 |
R. J. Gillespie, E. A. Robinson, Angew. |
|
Chem. 1996, 74, 1088–1096. |
|
Chem. Int. Ed. Engl. 1996, 35, |
30 |
M. E. Alikhani, Y. Bouteiller, B. Silvi, |
|
495–514. |
|
J. Phys. Chem. 1996, 100, 16092–16097. |

160 6 The ELF Topological Analysis Contribution to Conceptual Chemistry and Phenomenological Models
31 |
B. Silvi, A. Savin, F. R. Wagner, in |
|
McWeeny, Y. G. Smeyers, S. Wilson), |
|
Modelling of Minerals and Silicated |
|
Kluwer Academic Publisher, |
|
Materials (Eds. B. Silvi, P. D’Arco), |
|
Dordrecht, 2001 pp. 259–279. |
|
Kluwer Academic Publishers, |
50 |
J. Molina, J. Dobado, Theor. Chem. |
|
Dordrecht, vol. 15, topics in |
|
Acc. 2001, 105, 328–337. |
|
molecular organization and |
51 |
D. B. Chesnut, J. Comput. Chem. |
|
engineering ed., 1997 pp. 179–199. |
|
2001, 14, 1702–1711. |
32 |
S. Noury, F. Colonna, A. Savin, B. |
52 |
P. Fuentealba, A. Savin, J. Phys. |
|
Silvi, J. Mol. Struct. 1998, 450, 59–68. |
|
Chem. A 2001, 105, 11531–115336. |
33 |
R. Llusar, A. Beltra´n, J. Andre´s, S. |
53 |
H. Chevreau, I. Moreira, B. Silvi, F. |
|
Noury, B. Silvi, J. Comput. Chem. |
|
Illas, J. Phys. Chem. A 2001, 105, |
|
1999, 20, 1517–1526. |
|
3570–3577. |
34 |
A. Beltra´n, J. Andre´s, S. Noury, B. |
54 |
D. B. Chesnut, L. J. Bartolotti, Chem. |
|
Silvi, J. Phys. Chem. A 1999, 103, |
|
Phys. 2002, 278, 101–1102. |
|
3078–3088. |
55 |
D. B. Chesnut, Heteroatom Chem. |
35 |
S. Berski, B. Silvi, Z. Latajka, J. |
|
2002, 13, 53–62. |
|
Leszczynski, J. Chem. Phys. 1999, 111, |
56 |
S. Noury, B. Silvi, R. G. Gillespie, |
|
2542–2555. |
|
Inorg. Chem. 2002, 41, 2164–2172. |
36 |
D. B. Chesnut, A. Savin, J. Am. |
57 |
P. Mori-Sa´nchez, J. M. Recio, |
|
Chem. Soc. 1999, 121, 2335–2336. |
|
B. Silvi, C. Sousa, A. Martı´n Penda´s, |
37 |
I. Fourre´, B. Silvi, P. Chaquin, A. |
|
V. Luan˜a, F. Illas, Phys. Rev. B 2002, |
|
Sevin, J. Comput. Chem. 1999, 20, |
|
66, 075103. |
|
897–910. |
58 |
M. Feliz, R. Llusar, J. Andre´s, S. |
38 |
G. Frison, A. Sevin, J. Phys. Chem. A |
|
Berski, B. Silvi, New J. Chem. 2002, |
|
1999, 103, 10998–11003. |
|
26, 844–850. |
39 |
F. Fuster, B. Silvi, Theoret. Chem. Acc. |
59 |
M. Kohout, F. R. Wagner, Y. Grin, |
|
2000, 104, 13–21. |
|
Theor. Chem. Acc. 2002, 108, 150–156. |
40 |
B. Silvi, C. Gatti, J. Phys. Chem. A |
60 |
B. Silvi, F. Fuster, E. Kryachko, O. |
|
2000, 104, 947–953. |
|
Tishchenko, M. T. Nguyen, Molec. |
41 |
R. Choukroun, B. Donnadieu, J.-S. |
|
Phys. 2002, 100, 1659–1675. |
|
Zhao, P. Cassoux, C. Lepetit, B. Silvi, |
61 |
E. Chamorro, P. Fuentalba, A. Savin, |
|
Organometallics 2000, 19, 1901–1911. |
|
J. Comput. Chem. 2003, 24, 496–504. |
42 |
D. B. Chesnut, L. J. Bartolotti, Chem. |
62 |
B. Silvi, J. Pilme´, F. Fuster, E. M. |
|
Phys. 2000, 253, 1–11. |
|
Alikhani, in Metal–Ligand interactions |
43 |
D. B. Chesnut, L. J. Bartolotti, Chem. |
|
in molecular, nano, micro, and macro- |
|
Phys. 2000, 257, 175–181. |
|
systems in complex environments, |
44 |
T. Kato, H. Gornitzka, A. Baceiredo, |
|
NATO-ASI series (Eds. N. Russo, M. |
|
A. Savin, G. Bertrand, J. Am. Chem. |
|
Witko), Kluwer, Dordrecht, 2003 |
|
Soc. 2000, 122, 998–999. |
|
pp. 241–284. |
45 |
D. B. Chesnut, J. Phys. Chem. A 2000, |
63 |
J. Pilme´, B. Silvi, M. Alikhani, J. Phys. |
|
104, 7635–7638. |
|
Chem. A 2003, 107, 4506–4514. |
46 |
N. H. Werstiuk, H. M. Muchall, S. |
64 |
H. Chevreau, C. Martinsky, A. Sevin, |
|
Noury, J. Phys. Chem. A 2000, 104, |
|
C. Minot, B. Silvi, New J. Chem. 2003, |
|
11601–11605. |
|
27, 1049–1053. |
47 |
C. Fressigne´, J. Maddaluno, A. |
65 |
J. R. B. Gomes, F. Illas, B. Silvi, |
|
Marquez, C. Giessner-Prettre, J. Org. |
|
Chem. Phys. Lett. 2004, 388, 132– |
|
Chem. 2001, 65, 8899–8907. |
|
138. |
48 |
S. Berski, Z. Latajka, B. Silvi, J. |
66 |
R. J. Gillespie, S. Noury, J. Pilme´, B. |
|
Lundell, J. Chem. Phys. 2001, 114, |
|
Silvi, Inorg. Chem. 2004, 43, |
|
4349–4358. |
|
3248–3256. |
49 |
S. Berski, B. Silvi, J. Lundell, S. |
67 |
H. Chevreau, Chem. Phys. Lett. 2004, |
|
Noury, Z. Latajka, in New Trends in |
|
400, 59–61. |
|
Quantum Systems in Chemistry and |
68 |
L. F. Pacios, J. Phys. Chem. A 2004, |
|
Physics (Eds. J. Maruani, C. Minot, R. |
|
108, 1177–1188. |
|
|
|
References |
161 |
|
|
|
|
|
69 |
T. M. Klapotke, B. Krumm, K. |
88 |
J. Geier, H. Gru¨tzmacher, K. Exner, |
|
|
Polborn, I. Schwab, J. Am. Chem. Soc. |
|
H. Prinzbach, Angew. Chem. Int. Ed. |
|
|
2004, 126, 14166–14175. |
|
Engl. 2005, 44, 2433–2437. |
|
70 |
D. Chesnut, A. Crumbliss, Chem. |
89 |
F. Fuster, B. Silvi, Chem. Phys. 2000, |
|
|
Phys. 2005, 315, 53–58. |
|
252, 279–287. |
|
71 |
D. Chesnut, Chem. Phys. 2005, 315, |
90 |
F. Fuster, A. Sevin, B. Silvi, J. Phys. |
|
|
59–64. |
|
Chem. A 2000, 104, 852–858. |
|
72 |
F. Kraus, T. Hanauer, N. Korber, |
91 |
F. Fuster, A. Sevin, B. Silvi, J. Comput. |
|
|
Angew. Chem. Int. Ed. Engl. 2005, 44, |
|
Chem. 2000, 21, 509–514. |
|
|
7200–7204. |
92 |
X. Krokidis, S. Noury, B. Silvi, |
|
73 |
S. Shaiks, D. Danovich, B. Silvi, D. |
|
J. Phys. Chem. A 1997, 101, 7277– |
|
|
Lauvergnat, P. Hiberty, Chem. Eur. J. |
|
7282. |
|
|
2005, 21, 6358–6371. |
93 |
X. Krokidis, B. Silvi, M. E. Alikhani, |
|
74 |
J. Pilme, B. Silvi, M. E. Alikhani, |
|
Chem. Phys. Lett. 1998, 292, 35–45. |
|
|
J. Phys. Chem. A 2005, 109, |
94 |
X. Krokidis, V. Goncalves, A. Savin, |
|
|
10028–10037. |
|
B. Silvi, J. Phys. Chem. A 1998, 102, |
|
75 |
B. Gomez, N. V. Likhanova, M. A. |
|
5065–5073. |
|
|
Dominguez Aguilar, O. Olivares, J. M. |
95 |
X. Krokidis, B. Silvi, C. Dezarnaud- |
|
|
Hallen, J. M. Martinez-Magadan, |
|
Dandine, A. Sevin, New J. Chem. |
|
|
J. Phys. Chem. A 2005, 109, |
|
1998, 22, 1341–1350. |
|
|
8950–8957. |
96 |
X. Krokidis, N. W. Moriarty, W. A. |
|
76 |
D. Zubarev, A. Boldyrev, X. Li, L.-F. |
|
Lester, Jr., M. Frenklach, Chem. Phys. |
|
|
Cui, L.-S. Wang, J. Phys. Chem. A |
|
Lett. 1999, 314, 534–542. |
|
|
2005, 109, 11385–11394. |
97 |
X. Krokidis, R. Vuilleumier, D. |
|
77 |
W. Tiznado, O. B. Ona, V. E. Bazterra, |
|
Borgis, B. Silvi, Molec. Phys. 1999, 96, |
|
|
M. C. Caputo, J. C. Facelli, M. B. |
|
265–273. |
|
|
Ferraro, P. Fuentealba, J. Chem. Phys. |
98 |
S. Berski, J. Andre´s, B. Silvi, L. |
|
|
2005, 123, 214302. |
|
Domingo, J. Phys. Chem. A 2003, 107, |
|
78 |
I. Vidal, S. Melchor, J. Dobado, J. Phys. |
|
6014–6024. |
|
|
Chem. A 2005, 109, 7500–7508. |
99 |
M. C. Michelini, E. Sicilia, N. Russo, |
|
79 |
J.-F. Boily, T. M. Seward, Geochimica |
|
M. E. Alikhani, B. Silvi, J. Phys. |
|
|
et Cosmochimica Acta 2005, 69, |
|
Chem. A 2003, 107, 4862–4868. |
|
|
3773–3789. |
100 |
M. C. Michelini, N. Russo, M. E. |
|
80 |
J. Rosdahl, T. F. Fa¨ssler, L. Kloo, Eur. |
|
Alikhani, B. Silvi, J. Comp. Chem. |
|
|
J. Inorg. Chem. 2005, 14, 2888–2894. |
|
2004, 25, 1647–1655. |
|
81 |
C. Lepetit, B. Silvi, Chauvin, J. Phys. |
101 |
M. E. Alikhani, B. Silvi, J. Mol.Struct. |
|
|
Chem. A 2003, 107, 464–473. |
|
2004, 706, 3–6. |
|
82 |
C. Lepetit, V. Peyrou, R. Chauvin, |
102 |
V. Polo, J. Andres, R. Castillo, S. |
|
|
Phys. Chem. Chem. Phys. 2004, 6, |
|
Berski, B. Silvi, Chem. Eur. J. 2004, |
|
|
303–309. |
|
10, 5165–5172. |
|
83 |
J. C. Santos, W. Tiznado, R. |
103 |
V. Polo, J. Andre´s, J. Comp. Chem. |
|
|
Contreras, P. Fuentealba, J. Chem. |
|
2005, 26, 1427–1437. |
|
|
Phys. 2004, 120, 1670–1673. |
104 |
J. C. Santos, V. Polo, J. Andre´s, |
|
84 |
J. Herna´ndez-Trujillo, I. Garcı´a-Cruz, |
|
Chem. Phys. Lett. 2005, 406, 393– |
|
|
J. M. Martı´nez-Magada´n, Chem. Phys. |
|
397. |
|
|
2005, 308, 181–192. |
105 |
J. C. Santos, J. Andre´s, A. Aizman, P. |
|
85 |
F. Kraus, N. Korber, Chem. Eur. J. |
|
Fuentealba, V. Polo, J. Phys. Chem. A |
|
|
2005, 11, 5945–5959. |
|
2005, 109, 3687–3693. |
|
86 |
J. Poater, M. Duran, M. Sola`, B. |
106 |
A. Savin, R. Nesper, S. Wengert, T. F. |
|
|
Silvi, Chem. Rev. 2005, 105, 3911– |
|
Fa¨ssler, Angew. Chem. Int. Ed. Engl. |
|
|
3947. |
|
1997, 36, 1809–1832. |
|
87 |
K. Knabel, T. M. Klapo¨tke, H. No¨th, |
107 |
B. Silvi, I. Fourre´, E. Alikhani, |
|
|
R. T. Paine, I. Schwab, Eur. J. Inorg. |
|
Monatshefte fu¨r Chemie 2005, 136, |
|
|
Chem. 2005, 6, 1099–1108. |
|
855–879. |