
Matta, Boyd. The quantum theory of atoms in molecules
.pdf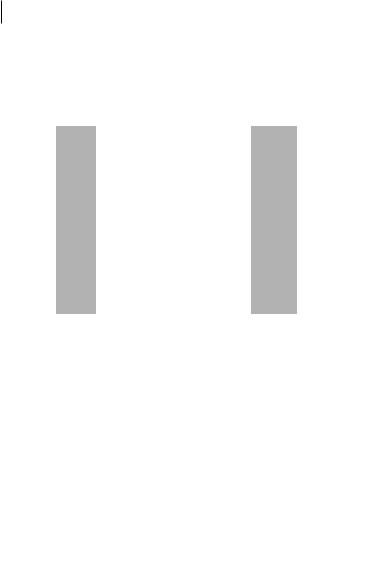
1325 Topological Atom–Atom Partitioning of Molecular Exchange Energy
Table 5.3 Exchange energy (kJ mol 1) between selected atomic basins (AM–BN, where M < N; Fig. 5.2) in (C2H2)2 from multipole expansion (increasing rank L) and 6D numerical integration (exact).
|
H2xC3 |
H2xH4 |
H2xC5 |
H2xH8 |
C3xH4 |
C3xH5 |
C3xC6 |
C3xH8 |
R (a.u.) |
4.27 |
6.28 |
8.03 |
6.23 |
2.01 |
7.48 |
9.72 |
5.49 |
|
|
|
|
|
|
|
|
|
L ¼ 1 |
45.9 |
1.5 |
0.1 |
0.3 |
1252.9 |
3.1 |
0.4 |
10.2 |
L ¼ 2 |
46.5 |
1.3 |
0.1 |
0.4 |
1638.9 |
4.2 |
0.5 |
14.1 |
L ¼ 3 |
42.1 |
1.3 |
0.1 |
0.4 |
1600.4 |
4.6 |
0.5 |
15.6 |
L ¼ 4 |
42.8 |
1.3 |
0.1 |
0.4 |
1455.8 |
4.7 |
0.5 |
16.2 |
L ¼ 5 |
44.2 |
1.3 |
0.1 |
0.4 |
1366.9 |
4.8 |
0.5 |
16.3 |
L ¼ 6 |
43.2 |
1.3 |
0.1 |
0.4 |
1398.6 |
4.8 |
0.5 |
16.3 |
L ¼ 7 |
42.8 |
1.3 |
0.1 |
0.4 |
1665.5 |
4.8 |
0.5 |
16.2 |
L ¼ 8 |
43.7 |
1.3 |
0.1 |
0.4 |
1973.7 |
4.8 |
0.5 |
16.2 |
L ¼ 9 |
43.5 |
1.3 |
0.1 |
0.4 |
1323.3 |
4.8 |
0.5 |
16.2 |
L ¼ 10 |
42.9 |
1.3 |
0.1 |
0.4 |
117.4 |
4.8 |
0.5 |
16.3 |
Exact |
43.3 |
1.3 |
0.1 |
0.4 |
1486.5 |
4.8 |
0.5 |
16.3 |
|
|
|
|
|
|
|
|
|
|
|
|
|
|
|
|
|
|
|
H4xC5 |
C5xC6 |
C5xH7 |
C5xH8 |
C6xH7 |
C6xH8 |
H7xH8 |
R (a.u.) |
8.05 |
2.26 |
4.27 |
2.01 |
2.01 |
4.27 |
6.28 |
|
|
|
|
|
|
|
|
L ¼ 1 |
0.1 |
3317.1 |
47.1 |
1222.7 |
1260.6 |
44.1 |
1.4 |
L ¼ 2 |
0.1 |
4062.3 |
47.5 |
1621.8 |
1646.8 |
45.2 |
1.3 |
L ¼ 3 |
0.1 |
3591.0 |
42.8 |
1580.8 |
1605.7 |
40.8 |
1.3 |
L ¼ 4 |
0.1 |
3782.0 |
43.7 |
1428.4 |
1461.3 |
41.2 |
1.3 |
L ¼ 5 |
0.1 |
5041.4 |
45.2 |
1358.2 |
1372.7 |
42.9 |
1.3 |
L ¼ 6 |
0.1 |
4568.9 |
44.1 |
1398.5 |
1399.6 |
42.1 |
1.3 |
L ¼ 7 |
0.1 |
761.7 |
43.6 |
1578.5 |
1689.4 |
41.1 |
1.3 |
L ¼ 8 |
0.1 |
1668.4 |
44.8 |
1940.6 |
2049.5 |
42.2 |
1.3 |
L ¼ 9 |
0.1 |
18659.7 |
44.5 |
1568.4 |
1264.1 |
43.0 |
1.3 |
L ¼ 10 |
0.1 |
23057.4 |
43.6 |
1528.1 |
466.0 |
41.3 |
1.3 |
Exact |
0.1 |
4003.1 |
44.2 |
1465.9 |
1493.0 |
42.0 |
1.3 |
|
|
|
|
|
|
|
|
gible. It is, again, gratifying to note that the hydrogen-bond exchange energy converges rapidly.
The exact and multipole-expanded exchange energies of fifteen selected atom– atom interactions in the ethyne dimer are presented in Table 5.3. The covalent bonds CaH and CcC do not converge, the exchange energy of the latter bond being roughly three times that of the former. The next largest values are approximately a factor of 30 smaller and occur between the non-bonded C and H atoms of the respective ethyne moieties. Very low rank expansions approximate the exact
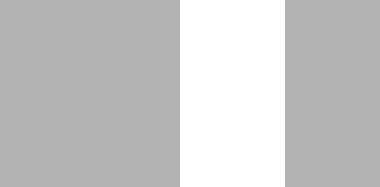
|
|
|
|
|
|
|
5.4 |
Results and Discussion |
133 |
||
Table 5.4 |
Exchange energy (kJ mol 1) between selected atomic basins |
|
|
|
|
||||||
|
|
|
|
||||||||
(AM–BN, where M < N; Fig. 5.2) in butane from multipole expansion |
|
|
|
|
|||||||
(increasing rank L) and 6D numerical integration (exact). |
|
|
|
|
|
||||||
|
|
|
|
|
|
|
|
|
|
|
|
|
|
C1xH4 |
C1xC5 |
C1xH6 |
C1xC8 |
C1xC11 |
H4xC11 |
C5xH6 |
C5xC8 |
|
|
R (a.u.) |
|
2.07 |
2.89 |
4.08 |
4.83 |
7.42 |
8.03 |
2.07 |
2.89 |
|
|
|
|
|
|
|
|
|
|
|
|
|
|
L ¼ 1 |
|
1219.3 |
906.4 |
27.8 |
27.0 |
4.2 |
0.3 |
1192.5 |
888.7 |
|
|
L ¼ 2 |
|
1531.9 |
1332.6 |
31.5 |
34.9 |
4.7 |
0.4 |
1509.1 |
1312.5 |
|
|
L ¼ 3 |
|
1598.9 |
1546.2 |
33.2 |
38.1 |
4.8 |
0.4 |
1579.9 |
1526.6 |
|
|
L ¼ 4 |
|
1478.5 |
1603.0 |
33.3 |
39.5 |
4.8 |
0.4 |
1476.2 |
1591.1 |
|
|
L ¼ 5 |
|
1363.2 |
1606.1 |
33.5 |
40.2 |
4.8 |
0.4 |
1368.3 |
1594.2 |
|
|
L ¼ 6 |
|
1356.8 |
1578.5 |
33.4 |
40.4 |
4.8 |
0.4 |
1358.1 |
1568.1 |
|
|
L ¼ 7 |
|
1531.2 |
1561.0 |
32.9 |
40.5 |
4.8 |
0.4 |
1456.9 |
1545.0 |
|
|
L ¼ 8 |
|
1731.5 |
1533.3 |
33.1 |
40.4 |
4.8 |
0.4 |
1588.1 |
1535.7 |
|
|
L ¼ 9 |
|
1569.5 |
1551.1 |
33.4 |
40.3 |
4.8 |
0.4 |
1626.0 |
1532.0 |
|
|
L ¼ 10 |
|
1446.1 |
1579.7 |
33.5 |
40.2 |
4.8 |
0.4 |
1515.3 |
1577.3 |
|
|
Exact |
|
1463.5 |
1568.1 |
33.2 |
40.4 |
4.8 |
0.3 |
1449.6 |
1556.0 |
|
|
|
|
|
|
|
|
|
|
|
|
|
|
exchange energies quite well. Several interactions, occurring between atoms more than 10 a.u. apart, for example H2–C6, H2–H7, C3–H7, and H4–C6 have vanishing exchange energy (i.e. <0.1 kJ mol 1). The convergent behavior of the expansion at short range is excellent, because the di erence of energy between ranks L ¼ 9 and L ¼ 10 is always less than 0.01 kJ mol 1 for non-bonded interactions and the maximum di erence between L ¼ 6 and L ¼ 10 is 0.13 kJ mol 1. The nonbonded interactions, for example C3–H8 and C3–C5, are not as strong as in the water dimer but are still significant in terms of ‘‘chemical accuracy’’ (1 kcal mol 1 or 4 kJ mol 1).
The exact and multipole-expanded exchange energies of eight selected atom– atom interactions in butane are shown in Table 5.4. As in the previous systems the convergence is faster than for the Coulomb interactions. Remarkably, for some bonded interactions (for example C5–C8) the di erence between the exact and L ¼ 10 is in the region of 20 kJ mol 1. Also, the exchange energy between C and H in covalent CaH bonds is fairly constant, whether in butane or ethyne.
The exact and multipole-expanded exchange energies of ten selected atom– atom interactions in 1,3,5-hexatriene are shown in Table 5.5. In this conjugated system the exchange energy can still be quite large over long distances. For example the exchange energy between the furthest atoms, C1 and C12, is 7.9 kJ mol 1. It is not clear why this value is larger in absolute magnitude than that for C1–C10, and appears as an exception in a regularly decreasing series of absolute energy values, starting with C1–C4. The same anomaly was found in the Coulomb interaction [26]. The formally CC double bonds, C1–C4 and C6–C8 are both associated with an energy of approximately 2:5 103 kJ mol 1. A single (sigma) CC bond, as found in butane (C1–C5, C5–C8), corresponds to
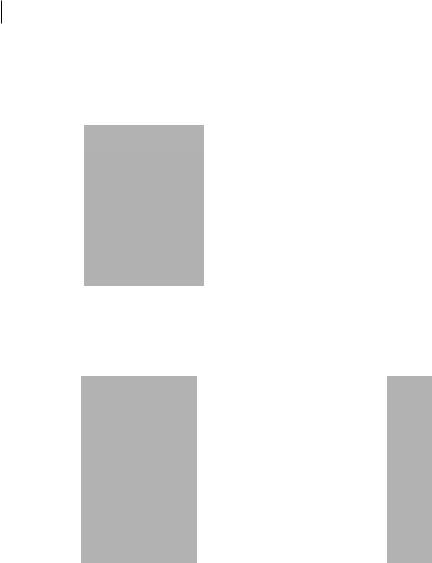
1345 Topological Atom–Atom Partitioning of Molecular Exchange Energy
Table 5.5 Exchange energy (kJ mol 1) between selected carbon basins (CM–CN, where M < N; Fig. 5.2) in 1,3,5-hexatriene from multipole expansion (increasing rank L) and 6D numerical integration (exact).
|
C1xC4 |
C1xC6 |
C1xC8 |
C1xC10 |
C1xC12 |
|
R (a.u.) |
2.53 |
4.66 |
|
6.99 |
9.32 |
11.60 |
|
|
|
|
|
|
|
L ¼ 1 |
1822.8 |
44.4 |
|
36.2 |
2.3 |
8.2 |
L ¼ 2 |
2458.4 |
54.0 |
|
36.1 |
2.3 |
8.0 |
L ¼ 3 |
2513.6 |
55.0 |
|
34.6 |
2.3 |
7.9 |
L ¼ 4 |
2536.0 |
54.8 |
|
34.7 |
2.3 |
7.9 |
L ¼ 5 |
2798.9 |
55.2 |
|
34.9 |
2.3 |
7.9 |
L ¼ 6 |
2916.1 |
56.1 |
|
34.9 |
2.3 |
7.9 |
L ¼ 7 |
2222.2 |
56.7 |
|
34.8 |
2.3 |
7.9 |
L ¼ 8 |
1346.2 |
55.7 |
|
34.8 |
2.3 |
7.9 |
L ¼ 9 |
3869.5 |
54.2 |
|
34.9 |
2.3 |
7.9 |
L ¼ 10 |
9335.7 |
55.7 |
|
34.8 |
2.3 |
7.9 |
Exact |
2605.6 |
55.5 |
|
34.9 |
2.3 |
7.9 |
|
|
|
|
|
|
|
|
C4xC6 |
C4xC8 |
|
C4xC10 |
C4xC12 |
C6xC8 |
R (a.u.) |
2.73 |
4.67 |
|
7.21 |
9.32 |
2.54 |
|
|
|
|
|
|
|
L ¼ 1 |
1102.9 |
41.8 |
|
4.9 |
2.3 |
1695.1 |
L ¼ 2 |
1625.7 |
51.7 |
|
5.4 |
2.3 |
2332.4 |
L ¼ 3 |
1821.3 |
52.9 |
|
5.4 |
2.3 |
2431.9 |
L ¼ 4 |
1863.8 |
52.6 |
|
5.4 |
2.3 |
2448.6 |
L ¼ 5 |
1892.1 |
53.0 |
|
5.4 |
2.3 |
2635.8 |
L ¼ 6 |
1909.8 |
54.0 |
|
5.4 |
2.3 |
2758.6 |
L ¼ 7 |
1803.0 |
54.4 |
|
5.4 |
2.3 |
2282.2 |
L ¼ 8 |
1599.5 |
53.6 |
|
5.4 |
2.3 |
1542.5 |
L ¼ 9 |
1816.2 |
52.5 |
|
5.4 |
2.3 |
3031.5 |
L ¼ 10 |
2759.3 |
53.6 |
|
5.4 |
2.3 |
7075.1 |
Exact |
1842.1 |
53.9 |
|
5.4 |
2.3 |
2498.6 |
|
|
|
|
|
|
|
approximately 1:5 103 kJ mol 1. The C4–C6 (hexatriene) exchange energy of1:8 103 kJ mol 1 could then be interpreted as, essentially, a single CC bond with some double bond character. We note that the Coulomb energy [24] of C1– C5 in butane is almost half that of C4–C6 in hexatriene. Hence it seems that the conjugation has much less e ect on the exchange energy than on the Coulomb
energy. |
8 |
|
|
The exact and multipole-expanded exchange energies of all |
¼ 28 atom– |
||
2 |
atom interactions in acrolein are shown in Table 5.6. Overall, excellent conver-
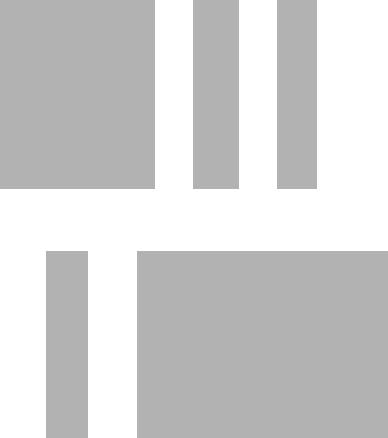
|
|
|
|
|
|
|
|
|
|
|
|
|
5.4 Results and Discussion |
135 |
||||
Table 5.6 |
Exchange energy (kJ mol 1) between all basins (AM–BN, |
|
|
|
|
|
|
|||||||||||
|
|
|
|
|
|
|||||||||||||
where M < N; Fig. 5.2) in acrolein from multipole expansion |
|
|
|
|
|
|
|
|||||||||||
(increasing rank L) and 6D numerical integration (exact). |
|
|
|
|
|
|
|
|
||||||||||
|
|
|
|
|
|
|
|
|
|
|
|
|
|
|
||||
|
C1xH2 |
C1xC3 C1xH4 |
C1xC5 |
C1xH6 |
C1xO7 |
|
C1xH8 |
H2xC3 |
H2xH4 H2xC5 |
|||||||||
R (a.u.) |
2.10 |
2.78 |
4.13 |
4.61 |
5.04 |
|
2.29 |
|
6.51 |
|
4.13 |
5.91 |
4.97 |
|
|
|||
|
|
|
|
|
|
|
|
|
|
|
|
|
|
|
|
|
|
|
L ¼ 1 |
1095.3 |
974.1 |
22.2 |
57.6 |
7.3 |
|
1692.7 |
5.0 |
|
34.6 |
4.8 |
7.5 |
|
|
||||
L ¼ 2 |
1461.1 1457.7 |
23.0 |
72.3 |
8.9 |
|
2145.5 |
5.1 |
|
35.1 |
3.8 |
9.8 |
|
|
|||||
L ¼ 3 |
1486.4 1659.2 23.1 |
72.1 |
9.2 |
|
2152.0 |
5.1 |
|
34.7 |
4.0 |
10.5 |
|
|
||||||
L ¼ 4 |
1377.5 1717.7 23.2 |
70.8 |
9.3 |
|
2184.3 |
5.1 |
|
34.6 |
4.0 |
10.8 |
|
|
||||||
L ¼ 5 |
1300.6 1731.6 23.5 |
72.2 |
9.4 |
|
2380.8 |
5.1 |
|
35.2 |
4.0 |
10.9 |
|
|
||||||
L ¼ 6 |
1337.3 1718.2 23.4 |
73.9 |
9.5 |
|
2464.4 |
5.1 |
|
35.1 |
4.0 |
10.9 |
|
|
||||||
L ¼ 7 |
1463.9 1651.5 23.2 |
73.6 |
9.5 |
|
2132.3 |
5.1 |
|
34.7 |
4.0 |
10.9 |
|
|
||||||
L ¼ 8 |
1496.8 1583.0 23.3 |
72.2 |
9.4 |
|
1659.0 |
5.1 |
|
34.9 |
4.0 |
10.9 |
|
|
||||||
L ¼ 9 |
1226.7 1695.7 23.4 |
72.3 |
9.5 |
|
2419.9 |
5.1 |
|
35.3 |
4.0 |
11.0 |
|
|
||||||
L ¼ 10 |
953.3 2001.9 23.3 |
73.9 |
9.5 |
|
4760.9 |
5.1 |
|
34.9 |
4.0 |
11.0 |
|
|
||||||
Exact |
1377.5 1687.8 23.1 |
73.1 |
9.6 |
|
2264.9 |
5.1 |
|
37.7 |
4.0 |
11.0 |
|
|
||||||
|
|
|
|
|
|
|
|
|
|
|
|
|
|
|
|
|||
|
|
|
|
|
|
|
|
|
|
|
||||||||
|
H2xH6 |
|
H2xO7 |
H2xH8 |
C3xH4 |
C3xC5 |
C3xH6 |
C3xO7 |
C3xH8 H4xC5 |
|
|
|||||||
R (a.u.) |
4.50 |
|
3.81 |
|
7.02 |
|
2.05 |
2.52 |
3.98 |
4.48 |
4.00 |
4.01 |
|
|
||||
|
|
|
|
|
|
|
|
|
|
|
|
|
|
|
||||
L ¼ 1 |
7.1 |
|
81.1 |
|
0.5 |
|
1205.7 |
1867.3 |
38.9 |
80.1 |
38.1 |
36.0 |
|
|
||||
L ¼ 2 |
9.9 |
|
78.7 |
|
0.6 |
|
1563.5 |
2494.7 |
39.3 |
97.6 |
39.6 |
37.8 |
|
|
||||
L ¼ 3 |
10.8 |
|
83.0 |
|
0.6 |
|
1586.7 |
2542.7 |
37.4 |
102.9 |
37.4 |
36.2 |
|
|
||||
L ¼ 4 |
10.9 |
|
82.2 |
|
0.6 |
|
1466.4 |
2578.3 |
37.8 |
104.3 |
37.7 |
36.3 |
|
|
||||
L ¼ 5 |
10.9 |
|
82.6 |
|
0.6 |
|
1354.3 |
2848.0 |
39.2 |
105.0 |
39.0 |
37.4 |
|
|
||||
L ¼ 6 |
10.9 |
|
83.3 |
|
0.6 |
|
1366.2 |
2926.2 |
38.6 |
106.1 |
38.5 |
36.9 |
|
|
||||
L ¼ 7 |
10.9 |
|
82.1 |
|
0.6 |
|
1594.6 |
2255.1 |
37.8 |
107.0 |
37.5 |
36.1 |
|
|
||||
L ¼ 8 |
10.9 |
|
82.4 |
|
0.6 |
|
1761.7 1521.3 38.6 |
106.1 |
38.2 |
36.8 |
|
|
||||||
L ¼ 9 |
10.9 |
|
83.3 |
|
0.6 |
|
1340.7 |
3844.3 |
39.1 |
104.6 |
39.1 |
37.5 |
|
|
||||
L ¼ 10 |
10.9 |
|
82.9 |
|
0.6 |
|
1015.4 |
8410.6 |
37.8 |
105.4 |
38.0 |
36.3 |
|
|
||||
Exact |
10.9 |
|
82.6 |
|
0.6 |
|
1462.0 |
2646.6 |
42.7 |
106.0 |
38.4 |
36.7 |
|
|
||||
|
|
|
|
|
|
|
|
|
|
|
|
|
|
|
|
|
|
|
gence rates are observed for acrolein. For all the nonbonded interactions there is a di erence between L ¼ 9 and L ¼ 10 of less than 2 kJ mol 1. The C1–C3 bond has an exchange energy equivalent to that of a single CC bond in butane and the p bond C5–C3 is also equivalent in energy to the C1–C4 double bond in 1,3,5- hexatriene. The CbO bond (C1–O7) has a similar exchange energy.
It is possible to establish a link with the delocalization index dðA; BÞ, which is interpreted as a bond order. One can prove that dðA; BÞ is proportional toEXABR, where R is the internuclear distance, if EXAB is restricted to L ¼ 1 (hence l1 þ l2 þ 1 ¼ 1 and therefore l1 ¼ l2 ¼ m1 ¼ m2 ¼ 0).
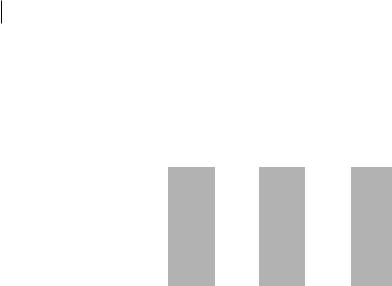
1365 Topological Atom–Atom Partitioning of Molecular Exchange Energy
Table 5.6 (continued)
|
H4xH6 |
H4xO7 |
H4xH8 |
C5xH6 |
C5xO7 |
C5xH8 |
H6xO7 |
H6xH8 |
O7xH8 |
R (a.u.) |
5.83 |
4.98 |
4.71 |
2.05 |
6.72 |
2.05 |
7.33 |
3.49 |
8.46 |
|
|
|
|
|
|
|
|
|
|
L ¼ 1 |
6.3 |
8.6 |
3.8 |
1223.3 |
36.7 |
1236.8 |
2.3 |
25.2 |
2.0 |
L ¼ 2 |
5.2 |
11.0 |
3.7 |
1576.5 |
36.4 |
1592.6 |
2.4 |
19.5 |
1.9 |
L ¼ 3 |
5.4 |
11.7 |
3.7 |
1601.0 |
35.4 |
1610.8 |
2.3 |
20.8 |
1.8 |
L ¼ 4 |
5.4 |
11.8 |
3.7 |
1474.8 |
35.5 |
1483.9 |
2.3 |
21.1 |
1.8 |
L ¼ 5 |
5.4 |
11.9 |
3.7 |
1363.7 |
35.6 |
1365.3 |
2.3 |
20.6 |
1.8 |
L ¼ 6 |
5.4 |
11.9 |
3.7 |
1375.0 |
35.5 |
1381.4 |
2.3 |
20.6 |
1.8 |
L ¼ 7 |
5.4 |
11.9 |
3.7 |
1641.6 |
35.5 |
1672.5 |
2.3 |
20.9 |
1.8 |
L ¼ 8 |
5.4 |
11.9 |
3.7 |
1837.3 |
35.5 |
1867.2 |
2.3 |
20.8 |
1.8 |
L ¼ 9 |
5.4 |
11.9 |
3.7 |
1173.7 |
35.5 |
1229.5 |
2.3 |
20.4 |
1.8 |
L ¼ 10 |
5.4 |
11.9 |
3.7 |
695.0 |
35.5 |
807.6 |
2.3 |
20.9 |
1.8 |
Exact |
5.37 |
11.9 |
3.7 |
1475.2 |
35.5 |
1485.6 |
2.3 |
20.7 |
1.8 |
|
|
|
|
|
|
|
|
|
|
5.4.2
Convergence of the Exchange Force
We computed the multipole expansion of the exchange force and compared it with the exact exchange force. Table 5.7 shows the results for some selected interactions of the water dimer. These two tables indicate that the convergence of the exchange force is not as good as the convergence of the exchange energy. For example, the average relative di erence in the interactions of acrolein (Table 5.8) considered is 2.7% for the magnitude of the force whereas the relative di erence of the energy for the same interactions is only 0.6%. In the water dimer the relative di erence reaches 7.6% for the force but only 1.0% for the energy.
5.4.3
Diagonalization of a Matrix of Exchange Moments
Coulomb multipole moments depend only on the electron density, but exchange moments explicitly refer to a set of molecular orbitals. The choice of these orbitals is arbitrary, up to a unitary transformation. This is why it is interesting to investigate the intrinsic information stored in the exchange moment matrix, Qlm, which is defined by the elements fQijlm; i ¼ 1; N; j ¼ 1; Ng where N is the number of molecular orbitals. We set out to diagonalize such a matrix and inspect its eigenvalues. For a given moment the corresponding matrix Qlm can be written:
N |
|
|
Qlmij ¼ Xk 1 |
UiklmqklmUjklm |
ð20Þ |
¼ |
|
|

|
|
|
|
|
5.4 |
Results and Discussion |
137 |
||
Table 5.7 |
Magnitude[a] of the exchange force between selected atoms |
|
|
|
|
||||
|
|
|
|
||||||
(AM–BN, where M < N; Fig. 5.2) for the water dimer computed from |
|
|
|
|
|||||
the multipole expansion and 6D integration. |
|
|
|
|
|
|
|||
|
|
|
|
|
|
|
|
|
|
|
O1xO4 |
O1xH5 |
H2xH3 |
H2xO4 |
H2xH5 |
H3xO4 |
H5xH6 |
||
R (a.u.) |
5.49 |
6.31 |
2.90 |
3.66 |
4.62 |
6.34 |
2.90 |
|
|
|
|
|
|
|
|
|
|
|
|
L ¼ 1 |
11.9 |
0.2 |
4.9 |
28.6 |
0.3 |
0.2 |
5.5 |
|
|
L ¼ 2 |
18.9 |
0.1 |
1.5 |
52.6 |
0.3 |
0.3 |
1.2 |
|
|
L ¼ 3 |
22.6 |
0.2 |
3.2 |
66.6 |
0.2 |
0.3 |
3.8 |
|
|
L ¼ 4 |
23.3 |
0.1 |
2.8 |
71.7 |
0.2 |
0.2 |
2.7 |
|
|
L ¼ 5 |
23.7 |
0.1 |
2.5 |
70.5 |
0.3 |
0.2 |
2.7 |
|
|
L ¼ 6 |
24.1 |
0.1 |
2.9 |
66.4 |
0.3 |
0.3 |
3.2 |
|
|
L ¼ 7 |
24.2 |
0.1 |
2.8 |
63.3 |
0.2 |
0.3 |
2.7 |
|
|
L ¼ 8 |
23.5 |
0.1 |
2.6 |
63.9 |
0.3 |
0.2 |
2.9 |
|
|
L ¼ 9 |
22.6 |
0.1 |
2.5 |
67.5 |
0.3 |
0.2 |
2.9 |
|
|
L ¼ 10 |
22.7 |
0.1 |
3.4 |
70.2 |
0.3 |
0.3 |
3.3 |
|
|
Exact |
23.4 |
0.1 |
2.7 |
66.6 |
0.3 |
0.2 |
2.9 |
|
|
|
|
|
|
|
|
|
|
|
|
a The magnitude of the force between the atoms H3 and H5 (R ¼ 7.38 au) is not shown because it is lower than 0.01 kJ mol 1 A˚ .
Table 5.8 Magnitude of the exchange force between selected atoms (AM–BN, where M < N; Fig. 5.2) for acrolein computed from the multipole expansion and 6D integration.
|
C1xH4 |
C1xC5 |
C1xH6 |
C1xH8 |
H2xH4 |
H2xH6 H2xO7 H2xH8 C3xO7 |
C3xH8 |
|||
R (a.u.) |
4.13 |
4.61 |
5.04 |
6.51 |
5.91 |
4.50 |
3.81 |
7.02 |
4.48 |
4.00 |
|
|
|
|
|
|
|
|
|
|
|
L ¼ 1 |
10.2 |
23.6 |
2.7 |
1.4 |
1.5 |
3.0 |
40.2 |
0.1 |
33.8 |
18.0 |
L ¼ 2 |
11.0 |
35.6 |
4.0 |
1.5 |
0.9 |
5.4 |
37.8 |
0.2 |
48.5 |
19.4 |
L ¼ 3 |
11.1 |
35.4 |
4.3 |
1.5 |
1.0 |
6.4 |
44.2 |
0.2 |
55.2 |
16.3 |
L ¼ 4 |
11.1 |
33.3 |
4.4 |
1.5 |
1.1 |
6.6 |
42.6 |
0.2 |
57.5 |
17.0 |
L ¼ 5 |
11.9 |
36.0 |
4.7 |
1.5 |
1.0 |
6.6 |
43.6 |
0.2 |
59.0 |
19.9 |
L ¼ 6 |
11.7 |
40.3 |
4.8 |
1.5 |
1.0 |
6.6 |
45.6 |
0.2 |
61.9 |
18.4 |
L ¼ 7 |
11.1 |
39.5 |
4.7 |
1.5 |
1.0 |
6.7 |
41.5 |
0.2 |
64.5 |
15.2 |
L ¼ 8 |
11.3 |
34.9 |
4.7 |
1.5 |
1.0 |
6.7 |
42.7 |
0.2 |
61.6 |
17.9 |
L ¼ 9 |
11.9 |
35.1 |
4.8 |
1.5 |
1.0 |
6.6 |
46.6 |
0.2 |
55.8 |
21.9 |
L ¼ 10 |
11.5 |
41.9 |
4.8 |
1.5 |
1.0 |
6.6 |
44.6 |
0.2 |
59.3 |
16.8 |
Exact |
11.2 |
37.8 |
4.9 |
1.5 |
1.0 |
6.7 |
43.5 |
0.2 |
60.9 |
17.9 |
|
|
|
|
|
|
|
|
|
|
|

1385 Topological Atom–Atom Partitioning of Molecular Exchange Energy
Table 5.9 Eigenvalues of the matrix of exchange moments Q00 for all atoms in the water dimer (labels as in Fig. 5.2).
n[a] |
O1 |
H2 |
H3 |
O4 |
H5 |
H6 |
1 |
2.0000 |
0.3725 |
0.4344 |
2.0000 |
0.4097 |
0.4103 |
2 |
1.9866 |
0.0182 |
0.0181 |
1.9795 |
0.0167 |
0.0168 |
3 |
1.9632 |
0.0151 |
0.0125 |
1.9315 |
0.0109 |
0.0109 |
4 |
1.6494 |
0.0107 |
0.0034 |
1.6315 |
0.0028 |
0.0028 |
5 |
1.5254 |
0.0010 |
0.0000 |
1.5262 |
0.0001 |
0.0001 |
6 |
0.0085 |
0.0005 |
0.0000 |
0.0223 |
0.0000 |
0.0000 |
7 |
0.0014 |
0.0002 |
0.0000 |
0.0039 |
0.0000 |
0.0000 |
8 |
0.0005 |
0.0000 |
0.0000 |
0.0020 |
0.0000 |
0.0000 |
9 |
0.0000 |
0.0000 |
0.0000 |
0.0000 |
0.0000 |
0.0000 |
10 |
0.0000 |
0.0000 |
0.0000 |
0.0000 |
0.0000 |
0.0000 |
Population |
9.1350 |
0.4182 |
0.4685 |
9.0970 |
0.4402 |
0.4409 |
|
|
|
|
|
|
|
a The number of the molecular orbital (accommodating 10 2 electrons in total), ranked from lowest to highest energy.
where fqklm; k ¼ 1; Ng are the eigenvalues of Qlm and the matrix Ulm represents the eigenvectors. Because the trace of the matrix is invariant with regard to the unitary transformation in Eq. (20), the trace of Qlm is equal to the sum of the eigenvalues.
Table 5.9 shows an example of such ‘‘exchange eigenvalues’’ qklm for each of the ten molecular orbitals and for each atom in the water dimer, when l ¼ m ¼ 0. An interesting property of this matrix is that its trace (i.e. the sum of its diagonal elements) is equal to the electrostatic multipole moments. This is illustrated for the water dimer, where the qk00 eigenvalues (of a given atom) summed over all molecular orbitals yield the population of that atom. The population of the water dimer amounts to 19.9998, illustrating the accuracy of the integration.
Using Eq. (20) we can now express the exchange energy with the diagonalized exchange moments:
Xl1 1 |
X2 2 |
|
N |
|
N |
|
||
XX |
ð21Þ |
|||||||
EX ¼ |
|
Tl1 m1 l2m2 |
¼ |
|
j |
¼ |
qil1m1 qjl2 m2 ðVijl1 m1 l2m2 Þ2 |
|
m |
l m |
i |
1 |
1 |
|
|||
|
|
|
where the matrix fVijl1m1l2m2 ; i ¼ 1; N; j ¼ 1; Ng is defined by:
Vl1m1l2m2 ¼ ðUAl1m1 ÞtUBl2m2 |
ð22Þ |
in which UlA1m1 and UlB2m2 are the eigenvectors of the atomic exchange matrices QlA1m1 and QlB2m2 . If Vl1m1l2m2 is the unit matrix the exchange energy is reduced to

References 139
the Coulomb energy and the exchange moments are equal to the electrostatic moments. As a result the eigenvector matrix Ulm characterizes the exchange nature of the interaction.
5.5 Conclusion
In this study we have focused on the exchange component of the Hartree–Fock energy or, more precisely, the ‘‘exchange-correlation’’ counterpart of the Kohn– Sham energy. In standard force fields this energy is absorbed in the parameterized bonded part, and not explicitly included in the non-bonded part. Our results show, however, that the mid-range intermolecular exchange energy is significant (tens of kJ mol 1) and is, therefore, a crucial part of the total energy. We presented the use of a spherical tensor multipole expansion to compute exchange energy and force for a set of small molecules and dimers. The multipole moments defined by the overlap of molecular orbitals converge well for the exchange and in many cases better than the Coulomb energy. The exchange force, however, seems to converge more slowly. The exchange energy associated with L ¼ 1 and multiplied by the internuclear distance is proportional to a previously established bond-order index.
References
1 |
W.D. Cornell, Cieplak P., Bayly C.I., |
7 |
S. Brodersen, Wilke S., Leusen F.F.J. |
|
Gould I.R., Merz K.M., Jr., Ferguson |
|
and G. Engel Phys.Chem.Chem.Phys. |
|
D.M., Spellmeyer D.C., Fox T., |
|
2003, 5, 4923–4931. |
|
Caldwell J.W., Kollman P.A. |
8 |
S.L. Price, Rev.in Comp.Chem. |
|
J.Am.Chem.Soc. 1995, 117, 5179– |
|
(Editors: K.B. Lipkowitz and D.B. |
|
5197. |
|
Boyd), Wiley, New York, USA, 2000. |
2 |
B.R. Brooks, Brucoleri R.E., Olafson |
9 |
S.L. Price, Harrison R.J., Guest M.F. |
|
B.D., Slater D.J., Swaminathan S., |
|
J. of Comp.Chem. 1989, 10, 552–567. |
|
Karplus M. J.Comp.Chem. 1983, 4, |
10 |
A.J. Stone, Price S.L. J.Phys.Chem. |
|
187–217. |
|
1988, 92, 3325–3335. |
3 |
W.R. Scott, Huenenberger P.H., |
11 |
G.M. Day, Motherwell W.D.S., Jones |
|
Tironi I.G., Mark A.E., Billeter S.R., |
|
W. Crystal Growth & Design 2005, 5, |
|
Fennen J., Torda A.E., Huber T., |
|
1023–1033. |
|
Krueger P., van Gunsteren W.F. |
12 |
W.T.M. Mooij, Leusen F.J.J. |
|
J.Phys.Chem. 1999, 103, 3596–3607. |
|
Phys.Chem.Chem.Phys. 2001, 3, |
4 |
J.W. Ponder, Case D.A. Adv. Protein |
|
5063–5066. |
|
Chem. 2003, 66, 27–85. |
13 |
V. Marcon, Raos G. J.Phys.Chem.B |
5 |
R.F.W. Bader, Atom in Molecules. A |
|
2004, 108, 18053–18064. |
|
Quantum Theory, Oxford Univ. Press |
14 |
E.R. Batista, Xantheas S.S., Jonsson |
|
(1990). |
|
H. J.Chem.Phys. 1999, 111, 6011– |
6 |
P.L.A. Popelier, Atoms in Molecules. |
|
6015. |
|
An Introduction, Pearson, London, |
15 |
C. Sagui, Pedersen L.G., Darden T.A. |
|
Great Britain (2000). |
|
J.Chem.Phys. 2004, 120, 73–87. |

140 5 Topological Atom–Atom Partitioning of Molecular Exchange Energy
16J.P. Ritchie, Copenhaver A.S. J.of Comp.Chem. 1995, 16, 777–789.
17U. Koch, Egert E. J.Comp.Chem. 1995, 16, 937–944.
18W.A. Sokalski, Keller D.A., Ornstein R.L., Rein R. J. of Comp.Chem. 1993,
14, 970–976.
19E.R. Batista J.Chem.Phys. 2000, 112, 3285–3292.
20D.S. Kosov, Popelier P.L.A.
J. Phys.Chem.A 2000, 104, 7339– 7345.
21D.S. Kosov, Popelier P.L.A.
J.Chem.Phys. 2000, 113, 3969–3974.
22P.L.A. Popelier, Rafat M.
Chem.Phys.Lett. 2003, 376, 148–153.
23M. Rafat, Popelier P.L.A. J.Chem.Phys. 2005, 123, 204103-1,7.
24P.L.A. Popelier, Joubert L., Kosov D.S.
J.Phys.Chem.A 2001, 105, 8254–8261.
25L. Joubert, Popelier P.L.A.
Phys.Chem.Chem.Phys. 2002, 4, 4353–4359.
26P.L.A. Popelier, Kosov D.S.
J.Chem.Phys. 2001, 114, 6539–6547.
27R. McWeeny, Methods of Molecular Quantum Mechanics, Academic Press, 2nd edn, SanDiego (1992).
28M. Rafat, Popelier P.L.A. J.Chem.Phys. 2006, 124, 144102-1,7.
29D.A. Varshalovich, Moskalev A.N., Khersonskii V.K., Theory of Angular Momentum, World Scientific (1988).
30A.J. Stone, The Theory of Intermolecular Forces, Clarendon, Oxford, Great Britain (1996).
31C. Haettig Chem.Phys.Lett. 1996, 260, 341–351.
32C. Haettig, Hess B.A. Molec. Phys. 1994, 81, 813–824.
33A.M. Pendas, Francisco E., Blanco M.A. J.Comp.Chem. 2005, 26, 344–351.
34X. Fradera, Austen M.A., Bader R.F.W. J.Phys.Chem. A 1999, 103, 304–314.
35J. Poater, Sola M., Duran M., Fradera X. Theor Chem Acc 2002, 107, 362–371.
36Y.G. Wang, Werstiuk N.H.
J.Comp.Chem. 2002, 24, 379–385.
37N.C. Handy, Schaefer III H.F.
J.Chem.Phys. 1984, 81, 5031–5033.
38A.D. Becke J.Chem.Phys. 1993, 98, 1372–1377.
39W. Koch, Holthausen M.C., A Chemist’s Guide to Density Functional Theory, Wiley–VCH, Weinheim, Germany (2000).
40GAUSSIAN03 Frisch, M.J.; Trucks, G.W.; Schlegel, H.B.; Scuseria, G.E.; Robb, M.A.; Cheeseman, J.R.; Montgomery, J.A.J.; Vreven, J.T.; Kudin, K.N.; Burant, J.C.; Millam, J.M.; Iyengar, S.S.; Tomasi, J.; Barone, V.; Mennucci, B.; Cossi, M.; Scalmani, G.; Rega, N.; Petersson, G.A.; Nakatsuji, H.; Hada, M.; Ehara, M.; Toyota, K.; Fukuda, R.; Hasegawa, J.; Ishida, M.; Nakajima, T.; Honda, Y.; Kitao, O.; Nakai, H.; Klene, M.; Li, X.; Knox, J.E.; Hratchian, H.P.; Cross, J.B.; Adamo, C.; Jaramillo, J.; Gomperts, R.; Stratmann, R.E.; Yazyev, O.; Austin, A.J.; Cammi, R.; Pomelli, C.; Ochterski, J.W.; Ayala, P.Y.; Morokuma, K.; Voth, G.A.; Salvador, P.; Dannenberg, J.J.; Zakrzewski, V.G.; Dapprich, S.; Daniels, A.D.; Strain, M.C.; Farkas, O.; Malick, D.K.; Rabuck, A.D.; Raghavachari, K.; Foresman, J.B.; Ortiz, J.V.; Cui, Q.; Baboul, A.G.; Cli ord, S.; Cioslowski, J.; Stefanov, B.B.; Liu, G.; Liashenko, A.; Piskorz, P.; Komaromi, I.; Martin, R.L.; Fox, D.J.; Keith, T.; Al-Laham, M.A.; Peng, C.Y.; Nanayakkara, A.; Challacombe, M.; Gill, P.M.W.; Johnson, B.; Chen, W.; Wong, M.W.; Gonzalez, C.; Pople, J.A. In Gaussian, Inc., Pittsburgh PA, 2003.

141
6
The ELF Topological Analysis Contribution to Conceptual Chemistry and Phenomenological Models
Bernard Silvi and Ronald J. Gillespie
6.1 Introduction
In the opinion of the celebrated mathematician and philosopher of science Rene´ Thom, chemical concepts such as those of bonds and valence lack scientific content [1]. He pointed out that the vocabulary used by chemists to describe matter at a microscopic level was often ambiguous. In fact most concepts have their origin in models developed at the beginning of the twentieth century and earlier which are, therefore, not fully consistent with quantum mechanics. To reconcile the chemical description of matter with the postulates of quantum mechanics it is necessary to build a mathematical model. This mathematical model is not unique, however, because di erent spaces (geometrical direct space, momentum space, Hilbert space) and di erent mathematical theories external to quantum mechanics can be used for this purpose.
The choice of the geometrical space has been pioneered by Raymond Daudel with the loge theory [2–4] the applicability of which remained limited to very small systems because the underlying mathematical theory, Shannon’s information theory [5], requires evaluation of the N-particle distribution function. The theory of dynamical systems [6, 7] is a powerful method of analysis of space which has been convincingly introduced in chemistry by Richard Bader with the quantum theory of atoms in molecules (QTAIM) [8]. This theory is based on analysis of the gradient field of the electron-density distribution which enables the partitioning of the molecular space into basins associated to each atom.
The purpose of this chapter is to provide a digest of the topological theory of chemical bonding with particular emphasis on epistemological aspects. QTAIM has made important contributions to conceptual chemistry, for example the definition of the atom within a molecule, of the bond critical point, of the bond path, and of the charge concentrations or depletions which emerge from analysis of the Laplacian of the charge density LðrÞ and which make a link with the valence shell electron pair repulsion (VSEPR) model [9–15]. To provide evidence for basins which correspond to bonds and lone pairs, however, one must perform dynamic