
Emerging Tools for Single-Cell Analysis
.pdfPotential Clinical Applications |
77 |
from residual tumor cells in the graft because of recent indications that many autografts are contaminated (Gazitt and Reading, 1996) before and after purging and because of evidence from gene-marking studies that residual (marked) tumor cells in an autograft are capable of contributing to relapse (Brenner et al., 1993; Deisseroth et al., 1994). Others believe that the number of tumor cells contained in an autograft is insignificant relative to residual disease in the host (Zhang et al., 1997). Only after reliable autograft purging methods are available will it be possible to determine whether the pretransplant therapy is effective in removing tumor from the host.
For example, high dose chemotherapy with autologous marrow or mobilized peripheral blood stem cell rescue has been extensively applied for the treatment of MM, resulting in improved event-free and overall survival when compared with standard chemotherapy (Attal et al, 1996; Barlogie et al., 1998). However, relapses are common and cure is unlikely in the majority of patients. Because both bone marrow and MPB are often contaminated with myeloma cells, it is conceivable that relapse after autotransplantation originates, at least in part, from the autografted tumor cells. Positive selection of CD34+ cells reduces the contamination of myeloma cells from the apheresis products up to 3 logs and provides a cell suspension capable of restoring normal hematopoiesis after a myeloablative conditioning regimen (Lemoli et al., 1998; Schiller et al, 1998). Nevertheless, there is considerable evidence that the lesion underlying the malignant B-cell proliferation observed in MM occurs in an immature precursor cell that expresses CD34. Purification of CD34+ Thy-1+ cells from MPB by flow cytometry has been shown to deplete myeloma cells by up to 7 logs, according to very sensitive flow cytometric analysis of CD38-bright cells and quantitative PCR analysis of patient-specific complementarity-determining region III (CDRIII) DNA sequences (Gazitt et al., 1995). Patient-specific oligonucleotide probes for the mutated CDRIII region in malignant B cells have been used to demonstrate elimination of residual tumor cells below detectable levels in the CD34+Thy-1+ subset, whereas tumor cells remain detectable in the CD34+Thy-1 subset (Reading et al., 1996). Therefore, CD34 selection alone is likely not sufficient for producing a tumor free graft in MM. Clinical trials to test the ability of CD34+Thy-1+ cells to provide hematopoietic support after myeloablative therapy for MM and other forms of cancer are ongoing. Preliminary results are presented later in this chapter.
Similarly, selection of CD34+ DR- cells from the bone marrow of chronic-phase CML (chronic myelogenous leukemia) patients has been shown to eliminate more BCR/ABL+ leukemic cells than merely selecting for CD34+ cells (Verfaillie et al., 1992; Leemhuis et al., 1993; Fogli et al., 1998). There is also limited evidence that tumor-propagating cells in certain types of NHL express CD34 (Macintyre et al., 1995), making it theoretically necessary to purify autografts further. Detection of residual disease is more difficult in NHL; therefore it is not known whether these tumor cells segregate into the CD34+Thy-1– population. It is known that purification of the CD34+Thy-1+ cell fraction from normal bone marrow results in the elimination of 5–6 logs of CD19+ B cells. Also, the lack of expression of Thy-1 on acute myeloid leukemia cells (Blair et al., 1997) makes selecting for CD34+Thy-1+ cells attractive as a potential therapy for the small number of AML clones that do not express CD34, but there have not been any clinical trials performed to confirm this hypothesis.
78 |
Application of High-Speed Sorting for CD34+ Hematopoietic Stem Cells |
Thus it seems likely that, for at least some cancers, autologous transplantation with highly purified CD34+Thy-1+ HSCs could result in the reinfusion of significantly fewer tumor-propagating cells than transplantation with CD34-selected autografts. It seems reasonable to believe that elimination of tumor cells from the graft in combination with more effective pretransplant antitumor therapy might result in clinical benefit. There may also be a clinical benefit associated with infusing highly purified HSCs after myeloablative conditioning into patients with severe autoimmune diseases because of the 4–6-log T-cell and B-cell) depletion associated with the HSC purification process. In general, the more rigorous the HSC purification process, the greater the associated T-cell depletion effect. Also, the lower the T-cell dose, the lower the probability of reinfusing autoreactive T-cells; therefore a highly purified HSC autograft should be superior to a CD34-selected autograft for treating autoimmune disorders. Typically, a purified HSC autograft containing approximately 1 × 106 HSCs/kg would contain a T-cell dose of only 1 × 103/kg. Clinical trials are required in order to test these hypotheses. Previous studies with marginally T-cell-depleted autografts have demonstrated safety, but efficacy has not been thoroughly assessed (Burt et al., 1998).
Likewise, the use of purified HSCs, essentially free of alloreactive T cells, B cells, and granulocytes, permits clinical studies aimed at eliminating graft-vs-host disease (GVHD) from occurring in HLA-mismatched allogeneic transplants. The low frequency of immunocompetent alloreactive donor T cells in a purified HSC allograft, compared to a CD34selected allograft, is likely to reduce the risk of GVHD but increase the risk of engraftment failure. Thus, sophisticated T-cell engineering studies are required, but perhaps more patients could receive potentially curative treatment if greater genetic disparity between host and allograft were tolerable.
Purified HSCs may also be superior to CD34+ cells as targets for gene therapy due to their self-renewal potential. Genetic modification of HSCs theoretically offers the chance of a lifetime cure, whereas the benefits of transducing a heterogeneous CD34 population may be short term. Once stable, long-term genetic modification of large numbers of HSCs is possible, diseases resulting from enzyme deficiencies, such as Factor VIII, adenosine deaminase, and glucocerebrosidase, may be cured through transduction of the appropriate normal genes into HSCs. There is also preliminary evidence to suggest that transduction of HIV resistance genes into HSCs may render progeny T cells resistant to infection (Bonyhadi et al., 1997).
Finally, the use of purified allogeneic HSCs for in utero transplantation offers an opportunity to treat and possibly cure severe combined immunodeficiency and certain genetic metabolic disorders while the fetus is still tolerant to genetic disparity. The original proof of principle was demonstrated with xenogeneic transplantation experiments that demonstrated engraftment of purified human HSCs in sheep fetuses (Srour et al., 1993; Zanjani et al., 1995). It is hoped that the disease pathology may improve after engraftment of normal stem cells that compete with defective host cells. It is especially important for this type of allograft to contain as few immunocompetent T cells as possible to avoid GVHD.
The ability to use high-speed cell sorting to isolate hematopoietic subpopulations from MPB and BM lends itself well, in theory, to stem cell transplantation therapy for
Potential Clinical Applications |
79 |
hematologic malignancy. The advantages are the high purity that can be achieved and the ability to select cells based on multiple cell surface markers simultaneously. The other selection devices being used to select CD34+ cells for transplantation can achieve almost as high a purity in much less time but can select for only one marker at a time and therefore do not offer the possibility of further defining the graft composition. The major disadvantages are the technical difficulty and the length of time required to purify clinically relevant doses of rare cell types. Whereas 5–10 million highly purified primitive hematopoietic stem cells are more than sufficient for most in vitro or even small-scale animal reconstitution studies, at least 20-fold higher numbers are required for testing hematopoietic reconstitution in human trials. Neither the minimum nor the optimal CD34+ or purified HSC dose has been established with certainty. Most studies suggest a dose range of 1–2 × 106 CD34+ cells/kg in order to ensure rapid granulocyte and platelet engraftment in an autologous setting and from 2 to 5 × 106 CD34+ cells/kg in an allogeneic setting (Bernstein et al., 1998; Zimmerman et al., 1994). Considering that primitive HSCs comprise between 10 and 60% of the CD34+ cell population, it would be reasonable to conclude that between 5 × 105 and 1 × 106 purified HSCs/kg would be sufficient for long-term engraftment. For most individuals, this translates to a target HSC dose of approximately 100 million cells. The HSC percentages can range from 0 to 2% in G-CSF mobilized peripheral blood and from 0 to 1% in bone marrow; therefore it remains a great technical challenge to use flow cytometry alone to purify these large numbers of cells. For cell types that comprise greater than 30% of the starting material, purifying 100 million cells would be feasible using flow cytometry directly, but whenever the starting percentage is low, as it is for CD34 , a preenrichment step is required prior to flow cytometric sorting. Otherwise, sort times become impractical. It could be done if sorting could somehow be automated so that multiple platforms are operating simultaneously, but even with “high speed sorting,” sort times would range from 150 to 300 h to isolate 100+ million CD34+ cells directly from G-CSF-mobilized peripheral blood. Examples of preenrichment steps are density gradient separation (Ferrero et al., 1998), centrifugal elutriation (Noga et al., 1986, 1991; Yoder et al., 1993), chemical depletion (Gazitt et al., 1995), and immunomagnetic separation (Ishizawa et al., 1993). Each has its own associated advantages and disadvantages. If judged solely on product purity and recovery, the immunomagnetic separation devices work very well, provided a highquality monoclonal antibody source is available.
Small-scale experiments using the various immunomagnetic CD34 selection devices can result in very high purities, with reasonable recovery, but there is often considerable variability in results. In a recent direct comparison study, CD34+ cells were enriched to a median purity of 92.2% (43.5–96.1% n = 17) with the Isolex device, 96.5% (66.6–99.2%, n = 17) with the MiniMACS, and 77.9% (31.4–93.6%, n = 15) with the Ceprate-LC. Median recovery of CD34+ cells was 30.8% (18.6–71.8%) for Isolex, 69.9% (39.1–100%) for MiniMACS, and 42.9% (23.7–100%) for Ceprate-LC (Kruger et al., 1998). A similar (unpublished) experiment at SyStemix performed with a clinically relevant (1 × 1010) starting population gave very similar results, except that the CD34 purity was lower (50%) after using the Ceprate system, and CD34 recovery from Isolex was above 80%.

80 |
Application of High-Speed Sorting for CD34+ Hematopoietic Stem Cells |
The introduction of technology that allowed for multiparameter sort decisions to be made at rates of up to 25,000 events/s, without compromising purity or recovery, has contributed to advances in our understanding of hematopoiesis by enabling various rare cell types to be isolated in sufficient numbers for animal and human transplant studies. The slower-speed, widely available commercial flow cytometers manufactured by Becton Dickinson (San Jose, CA) and Beckman-Coulter (Hialeah, FL) are best suited to the smaller research samples encountered on a daily basis in most institutions, since cell recovery, not sorting time, is the primary concern. The high-speed cell sorters currently manufactured by SyStemix (Palo Alto, CA) and by Cytomation (Fort Collins, CO) are best suited to sorting the large clinical-scale tissues, since their fluidics are designed for high throughput and they can make sort decisions about five-fold faster. The high-speed sorters do tend to have a higher setup-associated cell loss during calibration, but this is insignificant (~2%) when sorting a clinical-scale tissue. The two types of technology are complementary, since small-scale research studies allow investigators to make predictions and high-speed sorting enables them to test those predictions in animals and in humans.
Further improvement in sort rates is not likely without dramatic changes in fluidics design. Maximizing sort rates is dependent on the ability of the electronics to make sort decisions quickly and on the ability to pass cells through a laser beam, single file, at high speeds without exposing the cells to lethal pressure differentials. While there seems to be no limit to the potential for increasing the sort decision rate in the future, the physical trauma that the cells endure during the droplet generation process will likely prove to be the limiting factor for continued improvement. Not only is it hard to imagine viable cells surviving the increased stream velocity that would be required to take full advantage of faster electronics, but as the pressures and oscillation frequencies are being increased, it becomes more and more difficult to maintain a stable droplet break-off point. Future rate increases may eventually require use of a non-droplet-generating method such as that used in the optical sorter (Roslaniec et al., 1996, 1997).
SYSTEMIX PROCESS
For those diseases where rigorous and reproducible purification of HSCs is necessary to eliminate residual tumor cells or mature immunocompetent cells, flow cytometric cell sorting is the ideal method for end-stage purification, but it must follow one or more presort enrichment steps. SyStemix has developed a cell processing scheme that begins with shipping MPB apheresis products to a processing center and consists of CD34 enrichment, high-speed sorting for CD34+Thy-1+ cells, cryopreservation, shipment to the clinical site, thawing, and infusion. Since these cells are used for human transplantation studies, the manufacturing process as a whole, including the high speed sorting procedure, is compliant with current Good Manufacturing Practices (cGMPs) and with current U.S. FDA regulations governing industry-sponsored cellular therapy trials. Quality management is an integrated part of the manufacturing process.
SyStemix Process |
81 |
With mobilized peripheral blood, the “process” begins with the HSC mobilization in the patient and ends after the purified HSCs are reinfused back into the patient. Mobilization regimens are changing rapidly as new cytokines are found to be beneficial, but most often mononuclear cells (and HSCs) are harvested by apheresis following the fifth and sixth day of G-CSF administration (10 µg/kg/day). Alternatively, the combination of G-CSF or GM-CSF with chemotherapy (e.g., cytoxan, 4 g/m2) is useful for HSC mobilization in patients who have received multiple courses of prior chemotherapy. Following G-CSF mobilization, the apheresis product contains from 2 to 5 × 1010 cells and the HSC frequency ranges from 0.1 to 1%, whereas with G-CSF + cytoxan mobilization, each apheresis product typically contains between 0.1 and 1 × 1010 cells with the HSC frequency ranging from 1 to 5%. Depending on the degree of mobilization and the target number of HSCs, one to three aphereses are typically required to achieve the desired HSC dose. Before shipment to SyStemix, the individual MPB samples are diluted to a final concentration of less than 5 × 107 cells/ml with a proprietary transport medium. Once diluted, the cells can be stored (or shipped) for 24–36 h at 20–24°C without compromising HSC viability.
It is immediately apparent that it would be nearly impossible to sort such a large cell number (2 × 1010) single file through a flow cytometer, even with recent technological developments. Such a task would require approximately 300 h of continuous sorting at 20,000 events/s; therefore, some form of CD34 enrichment must be performed in advance. The combination of counterflow centrifugal elutriation and phenylalanine methyl ester (PME) lysis was originally used as the presort process to eliminate erythrocytes and mature myeloid cells prior to sorting for CD34+Thy-1+ cells. This presort process reduced the sample size by approximately 1 log, but the samples still required anywhere from 12 to 36 h of continuous sorting to process. For the last few years, SyStemix has utilized an immunomagnetic CD34 selection device to enrich for CD34+ cells prior to sorting for CD34+Thy-1+ cells. Whereas the elutriation/PME depletion method enriched CD34+ cells approximately 5-fold, the positive selection method enriches CD34+ cells approximately 40-fold. This reduction in presort sample size has resulted in a 4–8-h sorting process for each apheresis product and has thus greatly simplified the associated staffing issues.
The immunomagnetic CD34 selection process consists of four steps: (1) labeling cells with an anti-CD34 monoclonal antibody (MAb); (2) mixing cells with paramagnetic beads coated with sheep anti-mouse immunoglobulin G (IgG), whereby CD34+ cells form rosettes with the beads; (3) attaching the rosettes to a magnet and washing CD34– cells from the system; and (4) releasing the cells from the beads using a peptide mimotope that competes with the MAb binding site of the CD34 molecule. The process is designed to accommodate a wide variety of incoming tissues arriving from distant sites for processing in a reproducible, error-proof manner and requires approximately 6 h to perform. The process for isolating HSCs from BM is identical with that for MPB except that mobilization is not necessary, there is a bone marrow harvest instead of an apheresis procedure, and a buffy coat is prepared prior to CD34 selection.
Subsequently, the cells are stained with fluoresceinated MAbs directed against a different epitope of CD34 and against the Thy-1 antigen and are sorted on the high-

82 |
Application of High-Speed Sorting for CD34+ Hematopoietic Stem Cells |
speed clinical cell sorter (HSCCS). The purified HSC product is tested for purity, viability, and sterility and then cryopreserved using a controlled-rate freezer. Once the target dose is achieved and all products are released, the HSC infusion products are shipped to the clinical sites in a liquid nitrogen shipper and, upon arrival, returned to the liquid phase of liquid nitrogen until the time of infusion. Upon thaw, the cells are slowly diluted with 2 volumes of saline before reinfusion via an intravenous line.
REGULATORY AND QUALITY ASSURANCE ISSUES
Regardless of the cell type used, the FDA needs to approve the “manufacturing process” before any patients can be treated. The FDA published a draft “Points to Consider” document in 1991 that addressed their regulatory strategy for cell and gene therapy products. The traditional regulatory framework for pharmaceutical products was implemented for cell therapy products. Investigational New Drugs (IND) applications were required to initiate clinical trials, and manufacturing sites and processes were required to be licensed. The 1993 Federal Register Notice reinforced the requirement for conformity to existing regulations, including cGMPs. These standards were similar to the current quality assurance/good manufacturing practices defined for blood and tissue banks by the FDA in the late 1980s. They include organizational standards to ensure appropriate medical control of patient care and laboratory standards for collecting, processing, and storing patient tissues. In 1997, the FDA published another draft proposal for the regulation of celland tissue-based products, whereby the agency outlined distinct areas in which no FDA submissions were required and where FDA oversight would be limited. In this proposal, the FDA did not reduce its own authority to control the use of these cellular products, but it did remove some of the need for sites to be licensed to perform such manipulations. A distinction was drawn for autologous stem cell products that, when used for homologous purposes (e.g., hematopoietic reconstitution), do not require licensing by the FDA. The term “nonhomologous” was introduced to distinguish those products being altered in some way prior to infusion (ex vivo expansion and gene modification) and those products being used for purposes other than their natural function from the “minimally manipulated” cellular products, in terms of regulatory requirements. Details of the current and proposed regulations can be obtained by writing to the agency or via the Internet at www.FDA.gov.
Whether the FDA or individual professional societies hold ultimate responsibility for the regulation of these products, the resulting quality standards will likely resemble those imposed on traditional pharmaceutical products. Substantial emphasis is placed on control of the final product by requiring lot-to-lot release based on approved specifications for purity, identity, potency, and sterility. Exceptions to the rules will have to be granted on an individual basis due to the many inherent differences involved in producing a large quantity of a defined chemical product versus producing a small quantity of a living cellular product, but the “spirit” of the regulations will be very similar. Ultimately, each individual company is responsible for assuring that its product is both safe and effective for its intended use. Proving that each and every lot
Regulatory and Quality Assurance Issues |
83 |
manufactured meets preestablished quality standards consumes a significant percentage of the effort and cost required to produce a cellular therapy product. It is essential that a system be in place to ensure that all tissues are handled according to “good tissue practices” aimed at preventing contamination and preserving integrity and function. Most often, final product quality control testing is limited by the amount of product (i.e., the number of cells) available; therefore, a comprehensive quality assurance support system needs to be in place to supplement final product testing. For example, environmental monitoring programs and process validation experiments are crucial when there is an insufficient number of cells to test for sterility on a lot-to-lot basis.
In order to argue effectively that its products are 100% safe, a company must implement a quality assurance program that starts with proper facility and process design and includes equipment validation, raw material testing, documentation control, vendor qualification, personnel training, and internal auditing. Ideally, the manufacturing area is designed for handling potentially infectious agents in order to protect the products from cross-contamination and the personnel from the spread of infectious agents. The air-handling units within the facility should provide single-pass (100% outside) HEPA-filtered air at a slightly negative pressure relative to adjacent corridors. Separation of activities into separate processing suites with separate air-handling systems, gowning rooms, material flow patterns, cleaning stations, and waste flow patterns will ensure that cross-contamination does not occur. In order to maximize biosafety, disposable materials should be used for processing whenever possible. If reusable product- contact equipment is required, labor-intensive and expensive validation of all cleaning and sterilization procedures is necessary in order to prove that cross-contam- ination is impossible. It must also be kept in mind that these cellular products are only as sterile as the environments in which they are manufactured and that routine monitoring can be extremely useful in preventing accidental contamination. All open-con- tainer manipulations should occur in a class-100 biosafety cabinet that is disinfected between each use. Monitoring the air within the biosafety cabinet for microbial contamination during processing can result in data that support final product sterility claims. The document control procedures should force all manufacturing and quality control personnel to operate from the same set of current, peer-approved procedures. All raw material specifications, test methods, standard operating procedures, and manufacturing batch records should be controlled in a database capable of tracking the current status of each document. A copy of all relevant procedures is kept in the manufacturing or testing areas. All effective procedures require multidepartmental signature and significant justification for change. A history file is kept on each document so that it is possible to determine retrospectively which procedures were effective at the time a particular lot of material was manufactured.
Application of these quality assurance principles to a flow cytometric cell-sorting process is difficult, to say the least. These are not “black box” instruments designed for routine manufacturing use, especially when all fluidics, including the nozzle itself, need to be sterilized between different patients. They require talented, dedicated operators to keep them functioning reliably, and significant effort is required to verify in-process and final product quality. With these considerations in mind, SyStemix
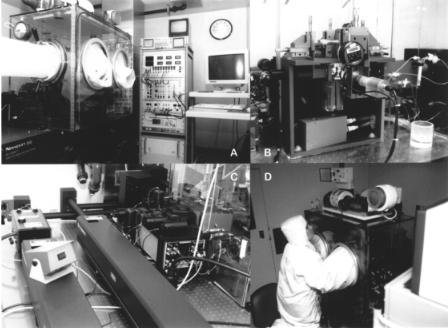
84 |
Application of High-Speed Sorting for CD34+ Hematopoietic Stem Cells |
F i g . 4.1. SyStemix high-speed clinical cell sorter. (A) Panoramic view of HSCCS, showing the optical table, isolator box with transfer and glove ports, electronics console, and computer interface. This is one of five instruments being used to purify human hematopoietic stem cells for clinical trials in the United States and France. (B) Close up of illumination chamber inside the isolator box from directly in front. Note disposable fluidics and chilled postsort sample storage capability. The camera provides a close-up view of the laser intercept and droplet break-off points. (C) View from the back of the instrument shows the current duallaser configuration. Efforts are underway to replace these with less expensive air-cooled lasers. (D) Side view of the HSCCS showing the operator at work fine tuning the alignment during the course of a sterile sort.
chose to design and build its own HSCCS for isolating clinically relevant numbers of HSCs. Although at least three commercially available cell sorters can now available that sort at relatively high acquisition rates, viable cell recovery, instrument sterilization, and containment of potentially pathogenic aerosols remain as obstacles to using those instruments to purify cells for clinical use.
The HSCCS pictured in Figure 4.1, is capable of sorting at rates exceeding 20,000 events/s. The instrument is based on the original design of van den Engh and Stokdijk (1989) at Lawrence Livermore National Laboratories but was modified extensively at SyStemix (Sasaki et al., 1995). SyStemix has used this instrument to purify CD34+Thy-1+ HSCs for stem cell transplants in breast cancer, MM, and NHL. A description of the unique technical and procedural considerations required for using this instrument for production of clinical trial material follows.
SyStemix’s HSCCS is a dual-laser instrument (488 nm primary and 633 nm secondary). The beams are individually focused to 40-µm spots that are spatially separated by 60 µm along the axis of the fluid jet. The detector table contains a photodiode for
Regulatory and Quality Assurance Issues |
85 |
FSC detection (Fig. 4.1B) and six photomultiplier tubes (Fig. 4.1C). With this configuration, up to five fluorescence (FL) signals (three primary and two secondary) may be detected simultaneously with two scatter signals. Bidirectional compensation between the FL1 and FL2 channels is built into the hardware. The instrument uses a commercially available nozzle assembly with a 70-µm tip (Becton Dickinson, San Jose, CA). A 10-l sheath tank is used to deliver phosphate-buffered saline (PBS) to the nozzle at a pressure of 44 psi for up to 30 hours of continuous, stable sorting. The nozzle assembly is oscillated at a frequency of 62 kHz, which provides a jet velocity of approximately 20 m/s and a stable droplet break-off at approximately 0.4 in. The instrument uses “off-the- shelf ” mirrors and filters for ease of repair and optical customization.
Sterilization of all product-contact instrument components is essential and is one of the major unique technical challenges that must be faced when sorting cells for clinical use. Sterilization protocols must be validated and are all encompassing. Detailed, written cleaning and sterilization procedures must describe ways to sterilize all reusable components of the fluidic assembly, calibration particles, and immediate cytometer environment surrounding the sorted cells.
The fluidics can either be sterilized by chemical exposure or else be purchased sterile and replaced between patients. Some investigators use 70% ethanol followed by copious amounts of either water or PBS to flush out the ethanol. SyStemix’s HSCCS was designed to utilize off-the-shelf medical tubing for easy access and replacement. The tubing is discarded and replaced between patients and is exposed to 30% hydrogen peroxide between multiple tissues from the same patient. Users of commercial cytometry equipment, such as the FACS Vantage (Becton Dickinson) or the Altra (Beckman-Coulter) are less able to routinely replace the tubing, since it is not easily accessed or available presterilized. In that situation, a chemical sterilization method could be developed, but this would require additional turn-around time between patients as well as the development and cost of tests to verify sterility and clearance of sterilant from the lines. While in-place sterilization is an option, it is certainly less convenient than the replacement system used on SyStemix’s HSCCS.
Reusable components such as sheath tanks, waste tanks, vial adapters, and nozzle assemblies need to be sterilized between patients. It is best if these components are designed to be autoclavable. For the sheath tanks in particular, filters can be attached to the air-in port as well as the sheath fluid-out ports prior to autoclaving. The nozzle assembly presents a somewhat more difficult problem. Few available nozzle assemblies can withstand repeated autoclave cycles. Some, such as the one used on the HSCCS, can withstand approximately five cycles but inevitably are damaged by the high heat and pressure of the autoclave. Others, such as the BD macrosort, contain the oscillation mechanism within the nozzle assembly itself and are less likely to withstand even those five cycles. Considering the replacement cost and effort required to validate a nozzle assembly for clinical use, an alternative sterilization method is required. SyStemix’s nozzle assemblies are sterilized by either radiation (e-beam) or hydrogen peroxide (H2O2). The latter can be labor intensive but is certainly less damaging than autoclaving. E-beam sterilization, on the other hand, is performed by an outside vendor, has a rapid turn-around time, and requires no in-house labor. The nozzle assemblies eventually wear out after repeated e-beam sterilization but have a mean time to

86 |
Application of High-Speed Sorting for CD34+ Hematopoietic Stem Cells |
failure of 15 cycles compared to 5 for autoclaving. SyStemix has validated that exposure to either H2O2 or e-beam radiation, used as circumstances require, provides sufficient sterilization for the nozzle assembly, whereas autoclaving is suitable for all the other reusable components. Once an appropriate cycle is developed, sterilization becomes a simple and well-established routine, but if any one of these components is not kept sterile, the sample, and therefore the patient, is put at risk.
Regarding reagents, it is of obvious importance to use only sterile reagents throughout the process. Some commercially available sheath fluids contain preservatives such as sodium azide that inhibit proliferation, but sterile, endotoxin-free Dulbecco’s phosphate-buffered saline (DPBS) can be either made in-house or purchased from a number of vendors and aseptically pumped into presterilized tanks. All calibration particles used to align the instrument need to be sterilized prior to use, since all previous sterilization efforts are nullified if a vial of contaminated beads is used to align the instrument. Sterile calibration particles are not readily available, but most commercial particles can be sterilized by mixing with H2O2. Although the process is time consuming, large quantities of calibration particles can be prepared at one time and transferred to single-use vials for later use.
The final sterilization issue to be considered is the immediate environment surrounding the tissue while it is being sorted. Although commercial cytometers are equipped with a flip-up or slide-closed sample chamber, they are difficult to sterilize and not likely to maintain sterility throughout the procedure. These difficulties inspired SyStemix to develop its own proprietary cytometer isolator system (Vardanega et al., 1997). This isolator completely encloses the sample area on the cytometer and thus protects both the tissue and the operator (Fig. 4.1A). Sterilization is accomplished via an H2O2 vapor generator (Steris, Mentor, OH). A pressure regulator on top of the isolator is fitted with incoming and outgoing HEPA filters (Fig. 4.1D) and maintains the isolator under slight positive pressure during the sort. The transfer unit on the left of the isolator fits to a transfer port on SyStemix’s customized biosafety cabinets and allows materials to be passed into the isolator under aseptic conditions. Users of commercial equipment would likely be required to either modify their cytometers for an isolator system or place the cytometer in a clean room in order to maintain the sterile environment required for clinical sorting.
IN-PROCESS AND FINAL PRODUCT TESTING
With high-speed sorting, any small error in calibration can have significant detrimental effects on the recovery of the target cell population; therefore, a battery of inprocess test procedures should be implemented to minimize this possibility. Because of the high pressures involved, a leak or a cracked line could cause significant loss of the patient sample within a matter of seconds, so all fluidic lines and components are stress tested immediately prior to sample introduction. Since the signal optimization and region determination steps can be very costly in terms of cell numbers when operating at high speeds, it can be helpful to analyze a small aliquot on a desktop analyzer, such as the FACSCalibur (Becton Dickinson), prior to analyzing the sample on the HSCCS. This instrument requires very few cells to determine approximate sort