
Astruc D. - Modern arene chemistry (2002)(en)
.pdf
Contents xi
10.1Introduction 330
|
Aims of this Account 330 |
10.2 |
The DoM Reaction as a Methodological Tool 332 |
10.2.1 |
The N-Cumyl Carboxamide, Sulfonamide, and O-Carbamate DMGs 333 |
10.2.2 |
The Lithio Carboxylate and Carboxylate Ester DMGs 334 |
10.2.3 |
The Di-tert-Butyl Phosphine Oxide DMG 336 |
10.3Heteroaromatic Directed ortho Metalation (HetDoM) in Methodological Practice 337
10.3.1 |
p-Excessive Heteroaromatic Directed ortho Metalation (HetDoM) |
337 |
|
10.3.1.1 |
Furans and Thiophenes 337 |
|
|
10.3.1.2 |
Indoles |
339 |
|
10.3.2 |
p-Deficient Heteroaromatic Directed ortho Metalation (HetDoM) |
342 |
|
10.3.2.1 |
Pyridines |
342 |
|
10.4The DoM–Transition Metal Catalyzed Aryl–Aryl Cross-Coupling Symbiosis 344
10.4.1 The Suzuki–Miyaura–DoM Link 345
10.4.2Aryl O-Carbamate and S-Thiocarbamate–Grignard Cross-Coupling
Reactions 346
10.4.3 The DoM–Negishi Cross-Coupling Connection 349
10.4.4DoM–Derived Cross-Coupling Reactions. Synthetic Comparison of Boron, Zinc,
and Magnesium Coupling Partners 350
10.5Beyond DoM: The Directed Remote Metalation (DreM) of Biaryl Amides and O-Carbamates – New Methodologies for Condensed Aromatics and
Heteroaromatics 351
10.5.1Heteroatom-Bridged Biaryl DreM. General Anionic Friedel–Crafts
|
Complements for Several Classes of Heterocycles |
356 |
10.6 |
Interfacing DoM with Emerging Synthetic Methods |
359 |
10.7 |
Closing Comments 362 |
|
|
References 363 |
|
11Arenetricarbonylchromium Complexes: Ipso, Cine, Tele Nucleophilic Aromatic Substitutions 368
Francoise Rose-Munch and Eric Rose
Abstract 368
11.1Introduction 368
11.1.1 |
E ects on Arene Reactivity of Cr(CO)3 Coordination |
368 |
|
||
11.1.2 |
Coverage and Definitions |
369 |
|
|
|
11.2 |
Ipso Nucleophilic Aromatic Substitutions 372 |
|
|
||
11.2.1 |
Carbon–Oxygen, –Sulfur and –Selenium Bond Formation |
372 |
|||
11.2.2 |
Carbon–Nitrogen and Carbon–Phosphorus Bond Formation |
378 |
|||
11.2.3 |
Carbon–Carbon Bond Formation |
383 |
|
|
|
11.2.4 |
Carbon–Hydrogen and Carbon–Metal Bond Formation 389 |
||||
11.3 |
Cine and Tele Nucleophilic Aromatic Substitutions |
392 |
|
||
11.3.1 |
Cleavage of CaF and CaCl Bonds |
392 |
|
|
|
11.3.2 |
Cleavage of CaO Bonds |
394 |
|
|
|
xii |
Contents |
|
|
|
|
|
Cleavage of CaN Bonds |
395 |
|
11.3.3 |
||||
11.4 |
Concluding Remarks |
396 |
||
|
|
Abbreviations 396 |
|
|
|
|
References |
397 |
|
12 |
Activation of Simple Arenes by the CpFeB Group and Applications to the Synthesis of |
|||
|
|
Dendritic Molecular Batteries 400 |
||
|
|
Didier Astruc, Sylvain Nlate, and Jaime Ruiz |
||
|
|
Abstract |
400 |
|
12.1Introduction 400
12.2 |
General Features of the CpFeþ Activation of Arenes |
401 |
12.2.1 |
Complexation and Decomplexation 401 |
|
12.2.2 |
Solubility, Stability, and General Reactivity Trends |
402 |
12.2.3 |
Single-Electron Reduction and Oxidation 403 |
|
12.2.4Deprotonation 403
12.2.5Reaction of the 19-Electron FeI Complex with O2: Extraordinary Reactivity of
|
Naked Superoxide and its Inhibition |
404 |
12.2.6 |
Nucleophilic Reactions 405 |
|
12.2.7 |
Heterolytic Cleavage of Aryl Ethers |
406 |
12.3CpFeþ-Induced Hexafunctionalization of Hexamethylbenzene for the Synthesis
of Metallo-Stars 406
12.4CpFeþ-Induced Octafunctionalization of Durene in the Synthesis of
Metallodendrimer Precursors 411
12.5CpFeþ-Induced Triallylation of Toluene and Reactivity of the Triallyl Tripod
Towards Transition Metals 413
12.6Nonaallylation of Mesitylene for the Synthesis of Dendritic Precursors of Large Metallodendrimers 414
12.7CpFeþ-Induced Activation of Ethoxytoluene in the One-Pot Synthesis of a Phenol Dendron by Triple-Branching and Synthesis of Organometallic Dendrons 419
12.8Convergent and Divergent Syntheses of Large Ferrocenyl Dendrimers with
Good Redox Stabilities 421
12.9Polyferrocenium Dendrimers: Molecular Batteries? 426
12.10Large Dendrimers Functionalized on their Branches by the Electron-Reservoir
|
[FeCp(h6-C6Me6)]þ Groups: A Molecular Battery in Action 428 |
|
12.11 |
Conclusion 429 |
|
|
References |
431 |
13 |
Charge-Transfer Effects on Arene Structure and Reactivity 435 |
|
|
Sergiy V. Rosokha and Jay K. Kochi |
|
|
Abstract |
435 |
13.1Introduction 435
13.2Mulliken’s Quantitative Description of Intermolecular (Charge-Transfer) Complexes 436
|
|
|
Contents |
xiii |
13.2.1 |
Short Theoretical Background 436 |
|
|
|
|
|
|
||
13.2.2 |
Quantitative Evaluation of Arenes as Electron Donors |
437 |
|
|
13.2.3 |
Spectral (UV/vis) Probe for the Formation of CT Complexes |
438 |
|
|
13.2.4 |
IR Spectroscopic Studies of Charge-Transfer Complexation |
442 |
|
|
13.2.5 |
Thermodynamics of Charge-Transfer Complexation |
443 |
|
|
13.3 |
Structural Features of Arene Charge-Transfer Complexes |
445 |
|
|
13.3.1 |
Bonding Distance of the Donor/Acceptor Dyad in Arene Complexes 446 |
|||
13.3.2 |
Relationship Between Hapticity and Charge Transfer in Arene Complexes 447 |
13.3.3E ect of Charge Transfer on the Structural Features of Coordinated
|
Arenes 448 |
13.3.3.1 |
Expansion of the Arene Ring 448 |
13.3.3.2 |
p-Bond Localization in the Arene Ring 449 |
13.3.3.3Loss of Planarity of the Arene Ring and the Transition from p- to s- Binding 451
13.4 |
Charge-Transfer Activation of Coordinated Arenes 452 |
|
13.4.1 |
Carbon–Hydrogen Bond Activation |
453 |
13.4.2 |
Nucleophilic/Electrophilic Umpolung |
455 |
13.4.3Modification of the Donor/Acceptor Properties of Coordinated Arene
Ligands 457
13.5CT Complexes as Critical Intermediates in Donor/Acceptor Reactions of
Arenes 460
13.5.1E ects of the Donor/Acceptor Interaction on the ET Dynamics of Arene
|
Donors 461 |
|
|
13.5.1.1 |
Steric Control of the Inner/Outer-Sphere Electron Transfer 461 |
|
|
13.5.1.2 |
Thermal and Photochemical ET in Strongly Coupled CT Complexes |
463 |
|
13.5.2 |
Electron-Transfer Paradigm for Arene Transformation via CT Complexes 465 |
||
13.5.3 |
Electron-Transfer Activation of Electrophilic Aromatic Substitution |
469 |
|
13.5.4 |
Structural Pre-organization of the Reactants in CT Complexes 470 |
|
|
13.5.5 |
CT Complexes in Aromatic Nitration and Nitrosation 472 |
|
|
13.6 |
Concluding Summary 475 |
|
|
|
References |
475 |
|
14 |
Oxidative Aryl-Coupling Reactions in Synthesis 479 |
|
|
|
Guillaume Lessene and Ken S. Feldman |
|
|
|
Abstract |
479 |
|
14.1Introduction 479
14.2 |
Mechanistic Overview 480 |
14.3 |
Oxidative Coupling Reactions with Hypervalent Iodine Reagents 484 |
14.4 |
Other Reagents for the Oxidative Coupling Reaction 495 |
14.4.1Iron(III) 495
14.4.2 Vanadium, Thallium, and Lead 499
14.4.3Copper(II) 504
14.4.4 Electrochemical Methods 509
14.4.5 Other Metals 510
xiv |
Contents |
|
|
|
|
|
|
513 |
|
14.4.6 |
Non-Metal Mediated Methods |
|
||
14.5 |
Phase-Supported Oxidants 515 |
|
||
14.5.1 |
Reagents Supported on Inorganic Materials |
515 |
||
14.5.2 |
Polymer-Supported Hypervalent Iodine Reagents 515 |
|||
14.6 |
Control of Atropisomerism |
517 |
|
|
14.6.1 |
Transfer of Chiral Information via the Molecular Backbone 518 |
|||
14.6.2 |
Oxidative Coupling of Two Chiral Molecules |
524 |
||
14.6.3 |
Stoichiometric Chiral Oxidation Reagents 524 |
|||
14.6.4 |
Catalytic Enantioselective Oxidative Coupling |
527 |
14.7Conclusion 534 References 535
15 Oxidative Conversion of Arenols into ortho-Quinols and ortho-Quinone Monoketals – A Useful Tactic in Organic Synthesis 539
Ste´phane Quideau
Abstract 539
15.1Introduction 539
15.1.1 How to Prepare ortho-Quinols and ortho-Quinone Monoketals 540
15.1.2Why Bother with ortho-Quinols and ortho-Quinone Monoketals? 542
15.1.2.1 |
Synthetic Reactivity of ortho-Quinols and ortho-Quinone Monoketals 542 |
15.1.2.2 |
Biosynthetic Implications of ortho-Quinols and ortho-Quinone Monoketals 543 |
15.1.2.3Biomechanistic Implications of ortho-Quinols and ortho-Quinone Monoketals 545
15.2 |
Oxidative Dearomatization of ortho-Substituted Arenols 546 |
||
15.2.1 |
Anodic Oxidation 546 |
|
|
15.2.2 |
Metal-Based Oxidative Activation |
548 |
|
15.2.3 |
Halogen-Based Reagents |
550 |
|
15.3 |
Synthetic Applications of ortho-Quinols and ortho-Quinone Monoketals 554 |
||
15.3.1 |
Diels–Alder Cycloadditions |
554 |
|
15.3.2 |
Photochemical Rearrangements |
561 |
|
15.3.3 |
Nucleophilic Substitutions and Additions 563 |
15.4Conclusion 568 References 568
16 Molecular Switches and Machines Using Arene Building Blocks 574
Hsian-Rong Tseng and J. Fraser Stoddart
Abstract 574
16.1Introduction 574
16.2 |
From Self-Assembling [2]Catenanes to Electronic Devices 575 |
|
16.3 |
A Hybrid [2]Catenane Switch 580 |
|
16.4 |
A Self-Complexing Molecular Switch |
581 |
16.5 |
Pseudorotaxane-Based Supramolecular Machines 582 |
|
16.6 |
[2]Rotaxanes and Molecular Shuttles |
583 |
16.7 |
The Evolution of Photochemically Driven Molecular Switches 589 |
|
16.8 |
Chemically Switchable Pseudorotaxanes 594 |

Contents xv
16.9 |
Molecule-Based XOR Logic Gate 596 |
16.10Conclusions 597 References 597
Index 600

xvi
List of Contributors
Didier Astruc
Laboratoire de Chimie Organique et Organome´tallique
UMR CNRS No. 5802
Universite´ Bordeaux I
F-33405 Talence Cedex France
Margaret M. Boorum
Department of Chemistry
Merkert Chemistry Center
Boston College
Chestnut Hill, MA 02467-3860
U.S.A.
Uwe H. F. Bunz
Department of Chemistry and Biochemistry
The University of South Carolina
Columbia, SC 29208
U.S.A.
Armin de Meijere
Institut fu¨r Organische Chemie Georg-August-Universita¨t Go¨ttingen Tammannstraße 2
D-37077 Go¨ttingen Germany
Franc¸ois Diederich
Laboratorium fu¨r Organische Chemie ETH Ho¨nggerberg
HCI, G 313 CH-8093 Zu¨rich Switzerland
Karl Heinz Do¨tz
Institut fu¨r Organische Chemie und Biochemie Universita¨t Bonn
Gerhard-Domagk-Straße 1 D-53121 Bonn
Germany
Ken S. Feldman
Department of Chemistry
Eberly College of Science
The Pennsylvania State University
152 Davey Laboratory
University Park, PA 16802-6300
U.S.A.
Rainer Haag
Institut fu¨r Organische Chemie Georg-August-Universita¨t Go¨ttingen Tammannstraße 2
D-37077 Go¨ttingen Germany
W. Dean Harman
Department of Chemistry
University of Virginia
Charlottesville, VA 22901
U.S.A.
Christian G. Hartung
Department of Chemistry
Queen’s University
Kingston, ON
K7L 3N6
Canada
John F. Hartwig
Department of Chemistry
Yale University
P.O. Box 208107
New Haven, CT 06520-8107
U.S.A.
Henning Hopf
Institut fu¨r Organische Chemie Technische Universita¨t Braunschweig Hagenring 30
D-38106 Braunschweig Germany
Joseph M. Keane
Department of Chemistry
University of Virginia
Charlottesville, VA 22901
U.S.A.
Jay K. Kochi
Department of Chemistry
University of Houston
University Park
Houston, TX 77204-5003
U.S.A.
Guillaume Lessene
Department of Chemistry
Eberly College of Science
The Pennsylvania State University
152 Davey Laboratory
University Park, PA 16802-6300
U.S.A.
Mogens Brøndsted Nielsen Laboratorium fu¨r Organische Chemie ETH Ho¨nggerberg
HCI, G 313 CH-8093 Zu¨rich Switzerland
Sylvain Nlate
Laboratoire de Chimie Organique et
Organome´tallique
UMR CNRS No. 5802
Universite´ Bordeaux I
F-33405 Talence Cedex
France
Ste´phane Quideau
Laboratoire de Chimie des Substances Ve´ge´tales Centre de Recherche en Chimie Mole´culaire Universite´ Bordeaux I
351, cours de la Libe´ration F-33405 Talence Cedex France
Eric Rose
Laboratoire de Synthe`se Organique et Organome´tallique
UMR CNRS 7611
Universite´ Pierre et Marie Curie Boite Postale 181
Tour 44 – 1er e´tage 4, Place Jussieu
F-75252 Paris Cedex 05 France
List of Contributors |
xvii |
|
|
Franc¸oise Rose-Munch
Laboratoire de Synthe`se Organique et Organome´tallique
UMR CNRS 7611
Universite´ Pierre et Marie Curie Boite Postale 181
Tour 44 – 1er e´tage 4, Place Jussieu
F-75252 Paris Cedex 05 France
Sergiy V. Rosokha
Department of Chemistry
University of Houston
Houston, TX 77204-5003
U.S.A.
Jaime Ruiz
Laboratoire de Chimie Organique et
Organome´tallique
UMR CNRS No. 5802
Universite´ Bordeaux I
F-33405 Talence Cedex
France
Lawrence T. Scott
Department of Chemistry
Merkert Chemistry Center
Boston College
Chestnut Hill, MA 02467
U.S.A.
Victor Snieckus
Department of Chemistry
Queen’s University
Kingston, Ontario K7L 3N6
Canada
Joachim Stendel Jr.
Institut fu¨r Organische Chemie und Biochemie Universita¨t Bonn
Gerhard-Domagk-Straße 1 D-53121 Bonn
Germany
J. Fraser Stoddart
Department of Chemistry and Biochemistry
University of California, Los Angeles
405 Hilgard Avenue
Los Angeles, CA 90095
U.S.A.
Akira Suzuki
Department of Chemistry and Bioscience
Kurashiki University of Science and the Arts
Kurashiki-shi, 712-8505
Japan
xviii |
List of Contributors |
|
|
Hsian-Rong Tseng |
Mark T. Valahovic |
|
||
|
Department of Chemistry and Biochemistry |
Department of Chemistry |
|
University of California, |
University of Virginia |
|
Los Angeles |
Charlottesville, VA 22901 |
|
405 Hilgard Avenue |
U.S.A. |
|
Los Angeles, CA 90095 |
|
|
U.S.A. |
|

1
Arene Chemistry : From Historical Notes to the
State of the Art
Didier Astruc
The History of Benzene
The history of benzene is one of the most intriguing in science. It started in 1825 with the isolation of benzene by Michael Faraday from the condensed phase of pyrolyzed whale oil. Its planar cyclic structure was first proposed in 1861 by the Austrian physicist and physical chemist Johann Josef Loschmidt [1–5]. However, it was only fully understood some 70 years later, around 1930, with the advent of the modern theories of aromaticity, i.e. the theory of molecular orbitals (Hu¨ckel’s theory) [6–8] and the theory of resonance [9–12].
Loschmidt published the cyclic planar structure of benzene together with those of 121 other arene compounds in a unique 54-page booklet entitled Konstitution-Formeln der organischen Chemie in geographischer Darstellung, which constituted a masterpiece of 19th century organic chemistry [1]. An abstract of this book was published by Herman Kopp in Liebigs Jahresbericht in 1861 [2]. Crucially, Loschmidt’s representation of benzene was very close to the present one.
Four years later, in 1865, August Kekule´ proposed another planar cyclic structure, but in which double bonds were alternating with single bonds. In his article published in the Bull. Soc. Chim. Fr. [13], Kekule´ briefly refers to Loschmidt’s formula in a single sentence ‘‘Elle me paraıˆt pre´fe´rable aux modifications propose´es par MM. Loschmidt et Crum-Brown.’’ [10] (It seems to me preferable to the modifications proposed by Loschmidt and Crum-Brown). The strength of Kekule´’s structure (original representation below) is that this type of formalism is still in use today for the representation of arenes because it shows the tetravalency of carbon.
Whereas Loschmidt’s work was not much publicized, Kekule´’s structure of benzene immediately became well known, criticized, and controversial. Various other structures were proposed as substitution on benzene was shown to be easier than addition, which conflicted with the cyclohexatriene structure. Claus and Dewar proposed alternative structures in 1867, and Claus’ formula was adopted by Koener in 1874.
Ladenburg pointed out that the Kekule´ structure does not account for the fact that there is only one ortho-disubstituted benzene as its fixed double bonds should give rise to two isomers. Thus, Ladenburg suggested a prismatic geometry, for which there would also only be three disubstituted isomers as found experimentally for benzene, whereas Kekule´’s cyclohexatriene structure implies four disubstituted isomers. In 1872, Kekule´ answered this
Modern Arene Chemistry. Edited by Didier Astruc
Copyright 8 2002 WILEY-VCH Verlag GmbH & Co. KGaA, Weinheim ISBN: 3-527-30489-4
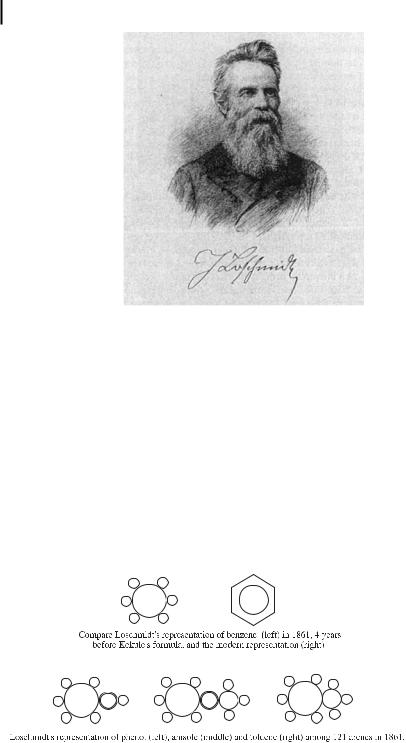
2Arene Chemistry : From Historical Notes to the State of the Art
Johann Josef Loschmidt (1821–1895) attended Prague University, and then at 21 went to Vienna to study first philosophy and mathematics, and then the natural sciences, physics and chemistry. After industrial ventures making potassium nitrate and oxalic acid among other products, he returned
to Vienna as a concierge in the early 1850s, and then became a school teacher. Always attracted by theoretical problems, he is also known for his calculation in 1865 of the number of molecules in one mL of gas (the ‘‘Loschmidt number’’). In 1866, he became Privatdozent at the University
of Vienna, was elected to the Royal Academy of Sciences in 1867, then became Associate Professor and got the honorary degree of Doctor of Philosophy in 1868. He founded the Society of Chemists and Physicists in Vienna (1869), became the Chairman of the Physical Chemistry Institute (1875), Dean of the Faculty of Philosophy (1877), and was elected to the Senate of the faculty (1885). He was a close friend of Josef Stephan and Ludwig Boltzmann, who were the greatest Viennese physicists of their time.