
Astruc D. - Modern arene chemistry (2002)(en)
.pdf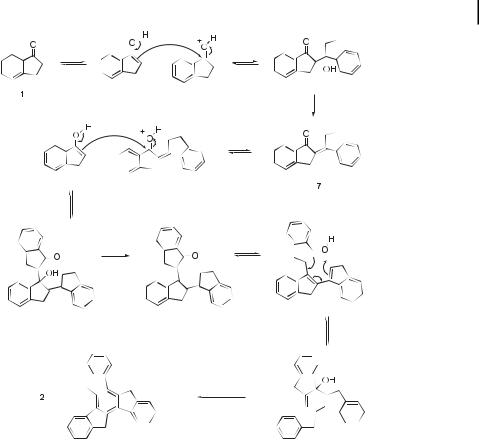
1.3 Other Examples 23
Fig. 6. Mechanism for the acid-catalyzed aldol trimerization of 1-indanone (1) to truxene (2).
polyphosphoric acid [18], polyphosphate ethyl ester [19], para-toluenesulfonic acid [20], and pyridinium hydrochloride [20]. While most of these reactions give truxene in good yield, the best synthesis appears to be that of Dehmlow [14], which is reported to give truxene in 98 % yield by heating 1 in a 2:1 mixture of hydrochloric acid and acetic acid at 100 C for 16 hours. The mechanism proposed for the acid-catalyzed trimerization of 1-indanone to truxene is outlined in Figure 6.
1.3
Other Examples
Truxene and truxone were the first members of what is now a large family of tris-annulated benzenes that have been synthesized by aldol trimerizations of cyclic ketones during the past 125 years. Table 1 provides some additional representative examples [6, 10, 21–29].
This list is undoubtedly incomplete; however, some general observations can be made on the basis of just these examples. First, it should be noted that good yields of cyclic trimers

24 1 The Synthesis of Tris-Annulated Benzenes by Aldol Trimerization of Cyclic Ketones
Tab. 1. Other representative cyclic ketones and their trimeric aldol condensation products.
Ketone |
Trimer |
Reaction Conditions |
Yield |
Ref. |
|
|
|
|
|
|
|
|
|
1. |
SiCl4 |
65 % |
21 |
|
|
2. |
HCl |
|
|
1. |
350 C, 2 h |
91 % |
22 |
2. |
HOAc, HCl(g) |
|
|
1. NaOH, 25 C, 4 d
2. H2SO4, MeOH, 1 h 64 % 23 3. 350 C, 1 h
1. NaOH, 25 C, 4 d
2. H2SO4, MeOH, 1 h 53 % 23 3. 350 C, 1 h
Conc. HCl |
not given |
18 |
100 C |
|
|
ZnCl2 |
not given |
18 |
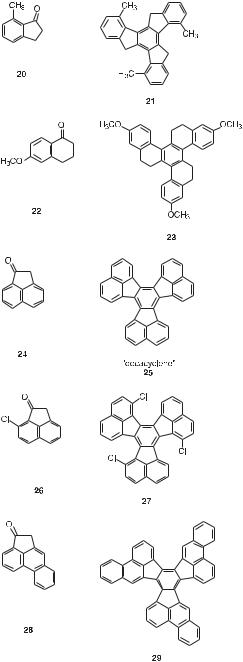
|
|
|
1.3 Other Examples |
25 |
||
Tab. 1. |
(continued) |
|
|
|
|
|
|
|
|
|
|
||
|
|
|
|
|
|
|
Ketone |
Trimer |
Reaction Conditions |
Yield |
Ref. |
||
|
|
|
|
|
|
|
|
|
TiCl4, Cl(CH2)4Cl |
14 % |
24 |
|
|
|
|
164 C, 30 min |
|
|
|
|
1. |
TiCl4, Et3N, 5 C |
|
|
2. |
25 C, 17 h |
41 % |
25 |
anthranilic acid |
not given |
26 |
heat |
|
|
TiCl4 |
|
|
o-C6H4Cl2 |
25 % |
6 |
180 C |
|
|
TiCl4 |
|
|
ClCH2CH2Cl |
95 % |
27, 28 |
83 C, 75 min |
|
|
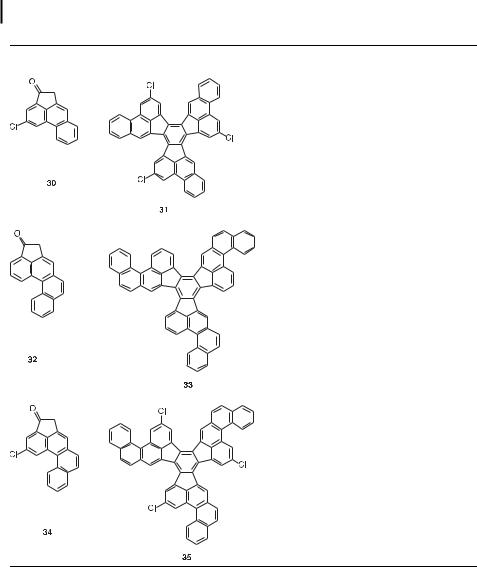
26 1 The Synthesis of Tris-Annulated Benzenes by Aldol Trimerization of Cyclic Ketones
Tab. 1. (continued)
Ketone |
Trimer |
Reaction Conditions |
Yield |
Ref. |
|
|
|
|
|
TiCl4
o-C6H4Cl2 83 % 28 100 C, 2 h
TiCl4 |
|
|
o-C6H4Cl2 |
80 % |
10, 28 |
100 C, 1 h |
|
|
TiCl4 |
|
|
o-C6H4Cl2 |
85 % |
28, 29 |
100 C, 2 h |
|
|
have been obtained not only with five-membered- but also with six-membered ring ketones, at least in some cases (e.g., cyclohexanone, 10).
It is equally noteworthy that both aliphatic and aromatic ketones undergo the aldol trimerization reaction.
For all but the most symmetrical trimers, two distinct cyclocondensations can be identified as potential synthetic routes starting from two isomeric ketones (e.g., 13 ¼ 15).
Finally, as one might expect, aldol trimerizations that lead to sterically crowded products generally fail or give the trimer only in modest yield (e.g., 21 and 27, the quoted yields for which represent optimized values). The high degree of regioselectivity seen in the trimeri-

1.4 Limitations 27
zation of 3-methylcyclohexanone (14) is another manifestation of this same e ect. We examine this limitation in more detail below.
1.4
Limitations
1.4.1
Experimental Observations and a Working Hypothesis
The 3,9,15-trichloro derivative of ‘‘decacyclene’’ (27, Table 1) has proven to be a superb precursor for the synthesis of ‘‘circumtriindene,’’ a bowl-shaped C36H12 PAH (geodesic dome) that constitutes 60 % of C60 [6]. Unfortunately, the aldol trimerization of 8-chloroacenaph- thenone (26, Table 1) gives the tris-annulated benzene 27 in only 25 % yield, even after extensive optimization of the reaction conditions [6]. It appears that the energetic cost of forcing three large chlorine atoms into the crowded fjord regions of the molecule diverts the reaction toward unwanted by-products. The extra strain energy contributed by the introduction of these three chlorine atoms to the decacyclene ring system is estimated to be about 17 kcal mol 1 on the basis of homodesmic density functional calculations [30, 31].
This steric e ect is even more dramatic in the next higher member of the series. Thus, whereas 4-acephenanthrenone (28) trimerizes to tribenzo[a,l,w]decacyclene (29) in almost quantitative yield under relatively mild conditions [27, 28], the chlorinated derivative 36 gives no more than traces of the cyclic trimer under a wide range of experimental conditions [28]. The only low molecular weight product isolated in the latter case (57 % yield þ recovered starting material) is the acyclic aldol dimer 37 (Figure 7). TiCl4-catalyzed aldol condensation of the bromo analogue of 36 likewise stops after the first step, giving the corresponding dimer in 95 % yield [28].
It did not go unnoticed in the above cases in which the trimerizations failed that the dimers obtained are the b,g-unsaturated enones and not the conjugated products that one might have expected to be thermodynamically more stable. Similar behavior had previously been observed for a-tetralone (38), which also gives a b,g-unsaturated enone in high yield rather than the cyclic trimer when treated with TiCl4 (Figure 8) [4].
In sharp contrast to these results, we obtained a pair of E- and Z-a,b-unsaturated ketones when the condensation of 28 was conducted under su ciently mild conditions [32] such
Fig. 7. 6-Chloro-4-acephenanthrenone (36) gives the b,g-unsaturated aldol dimer (37), which does not continue on to form the cyclic trimer; 6-bromo-4-acephenanthrenone behaves identically.
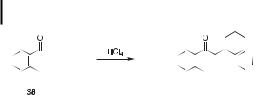
28 1 The Synthesis of Tris-Annulated Benzenes by Aldol Trimerization of Cyclic Ketones
Fig. 8. a-Tetralone (38) gives the b,g-unsaturated aldol dimer, which does not continue on to form the cyclic trimer.
that it terminated at the dimer stage [28]. Furthermore, careful examination of the 1H NMR spectra recorded during the condensation of 26 showed signals that could be attributed to two stereoisomeric conjugated enone intermediates. On the basis of these admittedly limited observations, we began formulating and scrutinizing the following empirical working hypothesis:
Formation of an a,b-unsaturated (conjugated) dimer from a cyclic ketone is vital to the success of an aldol trimerization reaction for the synthesis of a tris-annulated benzene; the reaction will fail with ketones that form only b,g-unsaturated (unconjugated) dimers.
In agreement with this hypothesis, a,b-unsaturated aldol dimers have been reported for many of the cyclic ketones discussed above that are known to proceed to tris-annulated benzene rings under appropriate cyclotrimerization conditions. Thus, in addition to the acenaphthenone derivatives 26 and 28 just mentioned, 1-indanone (2) and indane-1,3-dione (5), the precursors to ‘‘truxene’’ and ‘‘truxone’’ (Figures 2 and 3), have both been reported to give exclusively the a,b-unsaturated aldol dimers when the trimerization is suppressed [33, 34]. Because of symmetry, the latter (5) gives a single dimer, whereas the former (2) gives a pair of conjugated enones (E- and Z-isomers). Acenaphthenone itself (24) likewise gives only an a,b-unsaturated dimer (stereochemistry not determined) [35]. The aldol condensations of cyclopentanone (8) and cyclohexanone (10) can also be arrested at the dimer stage, and each of these ketones gives a mixture of both the a,b- and the b,g-unsaturated aldol dimers, with the conjugated enones dominating under equilibrating conditions [36]. Thus, we were gratified to find a considerable body of experimental data that is consistent with our hypothesis.
Are there any fundamental chemical principles that make this empirical hypothesis mechanistically reasonable? We believe there are. Looking back at Figure 6, one can identify two key bond-forming steps that bring together the three ketone molecules into a single acyclic trimer. Both steps are acid-catalyzed aldol condensation reactions, in which the enol of the starting ketone serves as the nucleophile. The electrophilic partners in these two steps are, respectively, the protonated monomer ketone (1) and the protonated dimer ketone (7). Obviously, any factor that favors the second of these two reactions (dimer ! trimer) over the first (monomer ! dimer) will set the stage for a cyclization and dehydration to the desired tris-annulated benzene. Conversely, if the first aldol step is fast relative to the second one, all of the monomer will be converted to the dimer, but there will then be no more monomer left to serve as the nucleophilic partner for the second aldol step, and the reaction will terminate at the acyclic dimer stage.
Since, at a given concentration of acid [37], a conjugated enone will be more extensively protonated than a ketone that lacks the extra a,b-unsaturation (e.g., 7 Hþ/7 vs. 1 Hþ/1), it is mechanistically reasonable that the dimer ! trimer step should be favorable for cyclic ketones that form a,b-unsaturated (conjugated) dimers. On the other hand, b,g-unsaturated al-
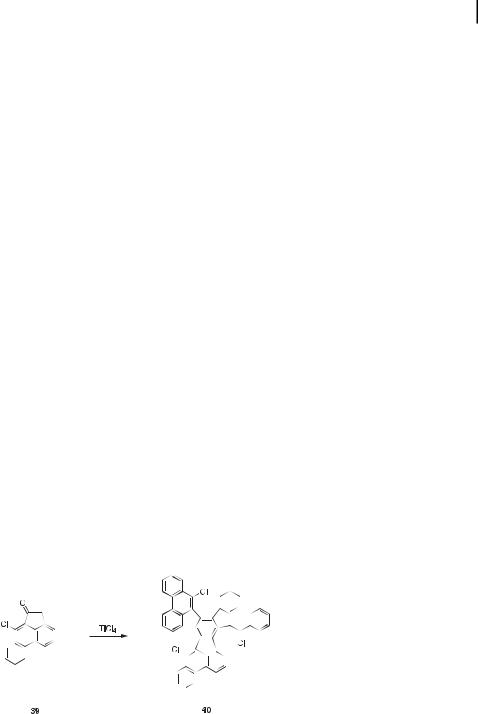
1.4 Limitations 29
dol dimers are less likely to make it all the way to the trimer because they are: (1) less fully protonated, and (2) more hindered than the starting ketone with which they must compete for the nucleophile. Thus, both halves of our hypothesis make sense.
To be useful, of course, a hypothesis must have predictive value. Can one predict in advance whether the aldol dimer from a particular ketone will be a,b-unsaturated or b,g- unsaturated?
1.4.2
Guidance from Calculations
We have performed single-point energy calculations, using density functional theory with AM1 optimized geometries (pBP/DN**//AM1) [31], on many of the ketones discussed above that are known to give tris-annulated benzenes by the aldol trimerization reaction (1, 5, 8, 10, 22, 24, 26, and 28). In agreement with our hypothesis, the a,b-unsaturated aldol dimer is calculated to be more stable than the b,g-unsaturated isomer in every case, sometimes by as much as 10 kcal mol 1 or more. In those cases in which the a,b-unsaturated aldol dimer can adopt either an E- or a Z-configuration, the more stable of the two was used for the comparison.
The tris-annulated benzene 40 is a particularly interesting example. As in many cases, one may envisage two ways of assembling this molecule by aldol trimerizations. We have attempted to prepare this obviously strained PAH derivative by subjecting both chloro ketones 36 and 39 to TiCl4 under a range of conditions [28]. The former gives almost exclusively the b,g-unsaturated dimer (37, Figure 7), with no more than traces of 40 being formed under any of the conditions we examined. The isomeric chloro ketone 39, on the other hand, gives significant quantities of 40 (Figure 9). Unfortunately, the yield of 40 obtained from the aldol trimerization of 39 is still too low to be synthetically useful, presumably due to steric factors at a later stage in the process. Nevertheless, this pair of reactions serves as an excellent illustration of the predictive power of our working hypothesis coupled with reliable energy calculations. At the pBP/DN**//AM1 level of theory, the most stable aldol dimer of chloro ketone 36 is the b,g-unsaturated isomer, which our hypothesis predicts will resist further condensation to give 40, and that is exactly what we observe. Conversely, calculations find the most stable aldol dimer for chloro ketone 39 to be the (Z )-a,b-unsaturated isomer, which our hypothesis identifies as a viable intermediate to continue on to 40, and this chloro ketone does indeed give the tris-annulated benzene 40.
Fig. 9. Aldol trimerization of 6-chloro-5-acephenanthrenone (39) to form a tris-annulated benzene. This behavior contrasts sharply with that of the closely related 6-chloro-4-acephenanthrenone (36) in Figure 7.

30 1 The Synthesis of Tris-Annulated Benzenes by Aldol Trimerization of Cyclic Ketones
The only exception that we have encountered so far to predictions based on this analysis is the aldol trimerization of a-tetralone (38). At the level of theory used above (pBP/DN**// AM1), the most stable aldol dimer is found to be the (E )-a,b-unsaturated isomer, which, according to our hypothesis, should condense further and cyclize to the tris-annulated benzene product. Experimentally, however, a-tetralone (38) gives a high yield of the b,g-unsaturated aldol dimer (Figure 8), which was calculated to be one of the less stable isomers. This ‘‘failure’’ must be either a shortcoming of our hypothesis or a shortcoming of the energy calculations. To test the latter possibility, we recalculated the energies of the aldol dimers of a- tetralone (38), still at the pBP/DN** level of density functional theory but this time with full geometry optimization at the pBP/DN** level of theory. These calculations required considerably more computer time than the original pBP/DN**//AM1 calculations, which use approximate geometries based on a fast semiempirical method (AM1), but at this higher level of theory the b,g-unsaturated aldol dimer of a-tetralone is found to be the lowest energy isomer. This lone ‘‘failure’’ can therefore be attributed to erroneous energy calculations, rather than to a flaw in the hypothesis. It is worth noting that, unlike a-tetralone, the 6-methoxy derivative of a-tetralone (22, Table 1), does yield a tris-annulated benzene (23) by the aldol trimerization reaction. In harmony with our hypothesis, the a,b-unsaturated dimer (E- isomer) of 22 is calculated to be more stable than the b,g-unsaturated dimer at the pBP/ DN** level of theory.
1.5
Conclusions
The aldol trimerization reaction has proven its value as a tool in organic synthesis. Many large PAHs can be assembled easily in one step from smaller, less complex starting materials by this method. For most unhindered cyclic ketones, there is rarely much difficulty with the first step (self-aldol) in the triple dehydration progression of monomer ! dimer ! trimer ! tris-annulated benzene (Figure 6). Likewise, the third step (cyclization– aromatization) is often very good (Figure 6), although steric impediments will generally lead to diminished yields. For this reaction cascade to succeed, however, the second step (crossaldol of the monomer ketone with the dimer ketone) must also be kinetically competitive. Herein, we have presented empirical evidence and mechanistic arguments to support the view that this requirement will be met only in those cases that lead to a,b-unsaturated (conjugated) dimers in the initial aldol condensation. Those ketones that give only b,g- unsaturated (unconjugated) dimers in the initial aldol condensation will not yield trisannulated benzenes (e.g., Figures 7 and 8).
In an attempt to derive some predictive power from this hypothesis, we have demonstrated how cases that are destined to fail at the second step can be identified in advance by relatively straightforward energy calculations on the isomeric aldol dimers. The number of examples is still limited, so one must use this hypothesis with caution, but we have so far found no exceptions.
Acknowledgements
We thank Stefan Hagen, Brandon J. McMahon, and John P. Amara for their contributions to our understanding of this chemistry and to the NSF for financial support.
|
|
|
References |
31 |
|
References |
|
|
|
|
|
|
|
|
1 |
D. Loguercio, Jr., Ph.D. Dissertation, |
21 |
S. S. Elmorsy, A. Pelter, K. Smith, |
|
|
UCLA, 1988. |
|
Tetrahedron Lett. 1991, 32, 4175–4176. |
|
2 |
C. Fabre, A. Rassat, C. R. Acad. Sci., Ser. |
22 |
S. V. Svetozarskii, G. A. Razuvaev, E. N. |
|
|
2 1989, 308, 1223–1228. |
|
Zil’berman, G. S. Volkov, J. Gen. Chem. |
|
3 |
F. Sbrogio, F. Fabris, O. De Lucchi, |
|
USSR 1960, 30, 2023–2027. |
|
|
Synlett 1994, 761–762. |
23 |
S. V. Svetozarskii, E. N. Zil’berman, |
|
4 |
S. Hagen, L. T. Scott, J. Org. Chem. 1996, |
|
G. A. Razuvaev, J. Gen. Chem. USSR |
|
|
61, 7198–7199. |
|
1959, 29, 1428–1431. |
|
5 |
L. T. Scott, M. S. Bratcher, S. Hagen, J. |
24 |
S. Hagen, L. T. Scott, unpublished |
|
|
Am. Chem. Soc. 1996, 118, 8743–8744. |
|
results. |
|
6 |
R. B. M. Ansems, L. T. Scott, J. Am. |
25 |
A. N. Pyrko, Zh. Org. Khim. 1992, 28, |
|
|
Chem. Soc. 2000, 122, 2719–2724. |
|
215–216. |
|
7 |
G. Mehta, G. Panda, P. V. V. S. Sarma, |
26 |
J. Moszew, W. Zankowska-Jasinska, |
|
|
Tetrahedron Lett. 1998, 39, 5835–5836. |
|
Roczniki Chemii 1958, 32, 225–233. |
|
8 |
M. Sarobe, R. H. Fokkens, T. J. Cleij, |
27 |
B. J. McMahon, B.Sc. Thesis, Boston |
|
|
L. W. Jenneskens, N. M. M. Nibbering, |
|
College, 1997. |
|
|
W. Stas, C. Versluis, Chem. Phys. Lett. |
28 |
M. M. Boorum, Ph.D. Dissertation, |
|
|
1999, 313, 31. |
|
Boston College, 2001. |
|
9 |
B. Gomez-Lor, O. de Frutos, A. M. |
29 |
L. T. Scott, M. M. Boorum, B. J. |
|
|
Echavarren, Chem. Commun. 1999, |
|
McMahon, S. Hagen, J. Mack, J. Blank, |
|
|
2431–2432. |
|
H. Wegner, A. de Meijere, Science, 2002, |
|
10 |
M. M. Boorum, Y. V. Vasil’ev, T. |
|
295, 1500–1503. |
|
|
Drewello, L. T. Scott, Science 2001, 294, |
30 |
Homodesmic reaction: |
|
|
828–831. |
|
25 þ 3(C6H5Cl) ! 27 þ 3(C6H6). |
|
11 |
M. J. Plater, Synlett 1993, 405–406. |
|
Calculation performed at the pBP/DN** |
|
12 |
S. Gabriel, A. Michael, Ber. Dtsch. Chem. |
|
level of theory with full geometry |
|
|
Ges. 1877, 10, 1551–1562. |
|
optimization using the Spartan collection |
|
13 |
W. Wislicenus, Ber. Dtsch. Chem. Ges. |
|
of programs [31]. |
|
|
1887, 20, 589–595. |
31 |
Spartan 5.0, Wavefunction, Inc., Irvine, CA. |
|
14 |
E. V. Dehmlow, T. Kelle, Synth. |
32 |
A. G. Holba, V. Premasager, B. C. |
|
|
Commun. 1997, 27, 2021–2031. |
|
Barot, E. J. Eisenbraun, Tetrahedron Lett. |
|
15 |
J. Hausmann, Ber. Dtsch. Chem. Ges. 1889, |
|
1985, 26, 571–574. |
|
|
22, 2019–2026. |
33 |
P. J. Williams, J. Chem. Soc., Chem. |
|
16 |
F. S. Kipping, J. Chem. Soc. 1894, 65, |
|
Commun. 1967, 719–720. |
|
|
269–289. |
34 |
K. Jacob, M. Sigalov, J. Y. Becker, A. |
|
17 |
F. S. Kipping, J. Chem. Soc. 1894, 65, |
|
Ellern, V. Khodorkovsky, Eur. J. Org. |
|
|
480–503. |
|
Chem. 2000, 2047–2055. |
|
18 |
R. Seka, W. Kellermann, Ber. Dtsch. |
35 |
J. Tatsugi, M. Okumura, Bull. Chem. Soc. |
|
|
Chem. Ges. 1942, 75, 1730–1738. |
|
Jpn. 1978, 51, 1227–1228. |
|
19 |
J. Chenault, C. Sainson, M. G. |
36 |
S. D. Mekhtiev, M. R. Musaev, A. G. |
|
|
Champetier, Comptes Rendus 1978, 287, |
|
Gasanov, Azerb. Khim. Zh. 1971, 93–97. |
|
|
545–547. |
37 |
Homodesmic reaction: |
|
20 |
T. W. Warmerdam, R. J. M. Nolte, W. |
|
7 þ 1 Hþ ! 7 Hþ þ 1 (DHcalc ¼ 13:5 |
|
|
Drenth, J. C. van Miltenburg, D. |
|
kcal mol 1). Calculation performed at the |
|
|
Frenkel, R. J. J. Zijlstra, Liquid Crystals |
|
pBP/DN**//AM1 level of theory using the |
|
|
1988, 3, 1087–1104. |
|
Spartan collection of programs [31]. |

32
2
Oligounsaturated Five-Membered Carbocycles – Aromatic and Antiaromatic Compounds in the Same Family
Rainer Haag and Armin de Meijere
Abstract
Most compounds containing oligounsaturated five-membered carbocycles ranging from cyclopentadiene to C20 fullerene display interesting electronic and structural properties. This chapter describes recent developments in this area, starting with stabilized cyclopentadienyl cations, then discusses several fulvenes as well as spiroannelated cyclopentadiene derivatives and eventually linearly as well angularly fused oligocyclic five-membered ring systems. Among these oligounsaturated di-, tri-, and oligoquinanes, the features of the rather unstable pentalene and acepentalene are discussed with respect to their generation and electronic ground states. Structural properties, including those of the stable dilithium diides as well as several metal complexes are presented. Finally, the generation and characterization of the highly unstable C20 fullerene, which sets a new benchmark for this class of molecules, are highlighted.
2.1
Introduction
The chemistry of oligounsaturated five-membered carbocycles has fascinated chemists for more than 100 years, since Thiele published the first synthesis of the aromatic cyclopentadienyl (Cp) anion 1 [1]. In the last couple of decades, knowledge about fully unsaturated oligoquinanes, the class of fused-ring compounds consisting of five-membered rings only, has experienced an exceptional growth (Figure 1) [2, 3]. Only recently has the existence of C20-fullerene 4, which is the smallest conceivable fullerene and can be considered as the Mount Everest of oligoquinanes, been proved [4]. This and many other new developments in the area of oligounsaturated five-membered carbocyclic compounds are highlighted in this chapter.
Cyclopentadienide (Cp) 1 is well known as one of the most frequently used ligands in organometallic chemistry. In addition, the cyclopentadienide anion 1 has always been quoted as a classic example of Hu¨ckel aromaticity, to demonstrate along with benzene and the cycloheptatrienyl cation the validity of the (4n þ 2) p-electron rule. In contrast, a simple and stable cyclopentadienyl cation of the type 1þ remains to be elusive [5]. With the highly unstable neutral cyclobutadiene and the cycloheptatrienyl anion, 1þ shares the character-
Modern Arene Chemistry. Edited by Didier Astruc
Copyright 8 2002 WILEY-VCH Verlag GmbH & Co. KGaA, Weinheim ISBN: 3-527-30489-4