
Astruc D. - Modern arene chemistry (2002)(en)
.pdf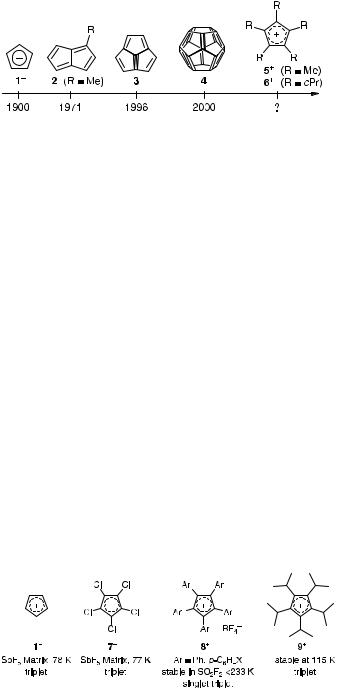
2.2 Cyclopentadienyl Cations |
33 |
|
|
Fig. 1. The non-linear time scale for the development of cyclopentadiene chemistry in the last century.
istics of possessing 4n p-electrons, and thus, according to Hu¨ckel MO theory, being antiaromatic. For higher oligoquinanes the situation is more complex, because Hu¨ckel theory is strictly valid only for monocyclic systems. Hence, reliable predictions concerning the electronic ground states are possible only on the basis of highest level ab initio calculations.
2.2
Cyclopentadienyl Cations
The parent cyclopentadienyl cation 1þ has been calculated at various levels of theory [6, 7], and has been found to have a triplet ground state. Its existence as a triplet species in an SbF5 matrix at 75 K had previously been proved by ESR spectroscopy [8]. Prior to that, the pentachlorocyclopentadienyl cation 7þ (Figure 2) had been generated, also in an SbF5 matrix at 77 K, and identified as a triplet on the basis of its observable ESR spectrum [9]. The pentaarylcyclopentadienyl cations 8þ [10], especially those with donor-substituted aryl substituents [11], are stable in solution up to 233 K, coexisting as singlet and triplet species due to the small energy di erence between them.
About 30 years later, the pentaisopropylcyclopentadienyl cation (9þ) was generated from the corresponding chloride and bromide and proved to be stable in the frozen solution at 115 K, existing as a triplet [12]. Pentaisopropylcyclopentadiene is unique in that it not only forms a stable anion – which is aromatic, just like any other cyclopentadienide – but also a persistent radical that has been characterized by ESR/ENDOR, 1H NMR spectroscopy, and mass spectrometry, as well as by X-ray crystal structure analysis [12].
Fig. 2. Previously known cyclopentadienyl cations.

34 2 Oligounsaturated Five-Membered Carbocycles -- Aromatic and Antiaromatic Compounds
What looked like a breakthrough towards the simple pentamethylcyclopentadienyl (Cp*) cation 5þ which was believed to be generated as the tetrakis(pentafluorophenyl)borate salt by hydride abstraction from pentamethylcyclopentadiene (10), [5a] soon turned out to be a misinterpretation of the X-ray crystal structure analysis. The observed structural characteristics of the obtained crystals, which were stable at room temperature for weeks, with two pyramidal carbon atoms in the five-membered ring, are simply those of a cyclic trimethylallyl cation 11 bridged by a slightly shortened single bond (1.48 A˚ ). [5b–e] The solid state 13C-NMR spectrum of 11 reflects the nonequivalence of the five ring carbons, with two signals (C2,5) in the cationic region (243 and 250 ppm) and that of C1 at 153 ppm. Thus, rather than undergoing a hydride abstraction to yield 5þ (RbMe), 10 had been protonated to give the cyclopentenyl cation 11 (Scheme 1).
Scheme 1.
Since the cyclopropyl group is well known to be a particularly good donor for electron-de- ficient centers, cyclopropyl-substituted cyclopentadienyl cations ought to be more stable than those with any other alkyl substituent. Indeed, the singlet pentacyclopropylcyclopentadienyl cation 6þ was predicted by DFT computations at the B3LYP/3-31þG** level of theory to be 19.4 kcal mol 1 more stable than the pentaisopropylcyclopentadiene singlet cation 9þ and 29.3 kcal mol 1 more stable than the Cp* singlet cation 5þ (Figure 3) [7].
The best potential precursors of the pentacyclopropylcyclopentadienyl cation 6þ have recently been prepared. The Grignard reagent 13, which was obtained by hydromagnesiation of easily accessible dicyclopropylacetylene (12), gave, upon reaction with butyl formate and
Fig. 3. Isodesmic stabilization energies of pentasubstituted cyclopentadienyl species (all structures fully optimized at the B3LYP/6- 31G* level).

2.2 Cyclopentadienyl Cations |
35 |
|
|
methyl cyclopropanecarboxylate – for the latter reaction after prior addition of cerium(III) chloride – tetracyclopropyl- (14) and pentacyclopropylcyclopentadiene (6), respectively, in good yields (Scheme 2) [13].
Scheme 2. (a) iBuMgBr (1 equiv.), Cp2TiCl2 (1–2 mol %), Et2O, 20 C, 30 min. – (b) Add inversely to HCO2nBu (0.9 equiv.) in THF, 20 C, 1 h. – (c) Add inversely to a suspension of CeCl3 (1.5 equiv.) in THF, 20C, 1 h. – (d) 1) Add cPrCO2Me in THF, 20 C, 1 h; 2) H2O/HOAc (6:1).
Upon treatment with an ethereal solution of methyllithium, both oligocyclopropylsubstituted cyclopentadienes 14 and 6 in tetrahydrofuran were quantitatively deprotonated to the corresponding cyclopentadienides 14-Li and 6-Li, respectively, which were characterized by their 1H and 13C NMR spectra. Treatment of the solutions of 14-Li and 6-Li with solutions of iron(II) chloride in tetrahydrofuran yielded the 1,10,2,20,3,30,4,40-octacyclopropylferrocene (16) (74%) and the decacyclopropylferrocene (17) (21%). After crystallization from hexane (for 16) and pentane/dichloromethane (for 17), the structures of both ferrocenes were established by X-ray crystal structure analyses (Scheme 3). The electron-donating e ect of the cyclopropyl substituents on these cyclopentadiene systems is manifested in the oxidation potentials of the ferrocenes 16 and 17. While the parent ferrocene has an oxidation potential E1=2 (vs. SCE) ¼ þ0:475 V, that of decamethylferrocene is significantly lower with E1=2 ¼ 0:07 V, and so are those of 16 (E1=2 ¼ 0:01 V) and 17 (E1=2 ¼ 0:13 V) [13].
Reaction of the cyclopentadienide 6-Li with N-chlorosuccinimide gave chloropentacyclopropylcyclopentadiene (19) in 95% yield. Hydride abstraction from 6-Li with triphenylmethyl tetrafluoroborate yielded the interesting tetracyclopropylcyclopropylidenecyclopentadiene 18 (37%). While the chloride 19, an ideal precursor to the cation 6þ, is stable at ambient temperature in the absence of moisture, it rearranges upon attempted chromatography on silica gel with the opening of one cyclopropyl ring to give the tetracyclopropylfulvene 20.
This rearrangement must occur by heterolytic cleavage of the carbon–chlorine bond in 19 to yield the intermediate cation 6þ, which is then attacked by the nucleophilic chloride at one of the partially positively charged cyclopropyl groups. With the less nucleophilic tetrafluoroborate counterion, generated by treating 19 with silver tetrafluoroborate under sol-

36 2 Oligounsaturated Five-Membered Carbocycles -- Aromatic and Antiaromatic Compounds
Scheme 3. (a) MeLi (1 equiv.) in Et2O, THF, 78 ! 20 C, 45 min. –
(b) Add to FeCl2 2THF in THF, 0 ! 20 C, reflux, 5 h. – (c) Ph3CBF4, THF. – (d) NCS, 0–20 C, 20 h. – (e) SiO2, pentane.
volytic conditions (in pentane/CH2Cl2/MeOH), 6þ BF4 survives even at 0 C and is trapped by methanol to give the methyl ether 21 without rearrangement in 75% yield (Scheme 4). The cation 6þ does indeed appear to be stable—at least at 115 C—in a non-nucleophilic solvent such as SO2ClF/SO2F2, when generated with a weakly coordinating counterion, e.g. by treatment of the chloride 19 with an excess of SbF5 [7]. The observed 13C-NMR signals are best interpreted with the singlet structure 6þ SbF6 (Scheme 4). Quenching the solution with methanol at 196 C and basic work-up at ambient temperature gave the methyl ether 21 in 40% yield.
Scheme 4. The relatively stable pentacyclopropylcyclopentadienyl cation 6þ SbF6 and the related fulvenyl cation 22þ BF4 .

2.3 Fulvene and Spiroannelated Cyclopentadiene Derivatives 37
The remarkable stabilizing e ect of the cyclopropyl substituents is also illustrated by the fact that the fulvene 18, upon protonation with tetrafluoroboric acid in dichloromethane, yields the fulvenyl tetrafluoroborate 22, which is completely stable in solution at 50 C. Compound 22 could be characterized by NMR spectroscopy (1H and 13C) and survives at ambient temperature for several days.
2.3
Fulvene and Spiroannelated Cyclopentadiene Derivatives
The chemistry of the so-called calicene 23 and its stable derivatives has recently been reviewed [14, 15], as has that of pentafulvalene 24 and its derivatives [14]. In view of the possible stabilization of at least the cationic moieties in the zwitterionic resonance structures 23þ and 24þ, it would certainly be interesting to prepare the percyclopropylated hydrocarbons 25 and 26. The easily accessible tetracyclopropylcyclopentadiene 14 [13] appears to be an appropriate precursor for these target molecules (Scheme 5).
Scheme 5.
Common to these molecules with their cyclopentadiene moieties is the so-called fulvene subunit 27. The first fulvenes, 6,6-dialkylfulvenes, were prepared as early as 1906 by Thiele et al. from sodium cyclopentadienide and ketones [16]. The parent hydrocarbon 27 and many other derivatives have been thoroughly studied since the 1960s [17–19]. Diazocyclopentadiene (28), which is also easily prepared from cyclopentadienide, is a heteroanalogue of fulvene. It has frequently been used as a precursor to other theoretically interesting molecules containing annelated cyclopentadiene moieties, because its irradiation readily generates the cyclopentadienylidene 29. This carbene has, for example, been trapped with alkynes to form spiro-annelated cyclopentadiene derivatives 30 (Scheme 5) [20]. It has been proved by UV spectroscopy [21] and supported by calculations [22] that these spiro[2.4]heptatrienes (so-called [1.2]spirenes) 30 experience a special kind of electronic
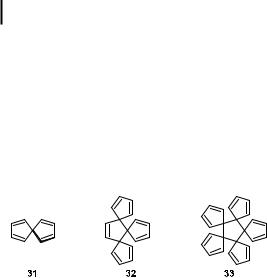
382 Oligounsaturated Five-Membered Carbocycles -- Aromatic and Antiaromatic Compounds
interaction, so-called spiroconjugation, across their central sp3 carbon atom in spite of the orthogonal orientation of the two p-systems.
As far as purely five-membered carbocyclic systems are concerned, spiro[4.4]nonatetraene (31) is the prototypical example of two p-systems experiencing electronic interaction through their common central carbon atom. Based on symmetry considerations, it was proposed that spiroconjugation in molecules such as 31 should result in a splitting of the cyclopentadienyl HOMOs, whereas the LUMOs should be degenerate [22, 23]. As a consequence, the first electronic absorption (EA) band of cyclopentadiene in 31 was predicted to be split into a lower and a higher lying component of quite di erent absorptivities.
Rather recently, the electronic structure of spiro[4.4]nonatetraene (31), as well as those of its radical anion and radical cation, respectively, have been probed by a variety of spectroscopic methods (electron transmission and energy loss in the gas phase, electronic absorption in cryogenic matrices) [24]. The extension of this concept of spiroconjugation to higher analogues of spiro[4.4]nonatetraene 31 would lead to the hydrocarbons 32 and 33. However, no attempts to prepare these compounds have yet been reported.
2.4
Polyunsaturated Di-, Tri-, and Oligoquinanes
Fully unsaturated fused-ring compounds, consisting only of five-membered rings, contain the most highly strained p-systems conceivable. Such unsaturated diand triquinanes and higher oligoquinanes are therefore unlikely to be found in natural sources, although saturated linear and angular triquinanes and higher oligoquinanes are known skeletons of naturally occurring sesquiterpenes. In addition to their high strain, these polyenes possess interesting electronic properties [25]. Although several groups have been and still are engaged in e orts to synthesize some of these fully unsaturated oligoquinanes [2], only three members of the series, 2 [26, 27], 3 [28], and 4 [4], have as yet actually been prepared or at least spectroscopically characterized as short-lived transients. Other members of the series have been approached synthetically, but the increasing ring strain in the series from the bicyclic pentalene 2 to C20-fullerene 4 makes the higher members less and less likely to be even observable [2].
Formally, higher oligoquinanes can be derived from fulvene (27) by the consecutive addition of etheno bridges. This concept has actually been successfully applied in the synthesis of the antiaromatic pentalene 2 [27], but it is unlikely to be practicable for higher members of this family due to the drastic increase in ring strain. While fulvene (27) and pentalene 2 possess almost planar geometries [2], acepentalene (3) and higher fully unsaturated oligo-
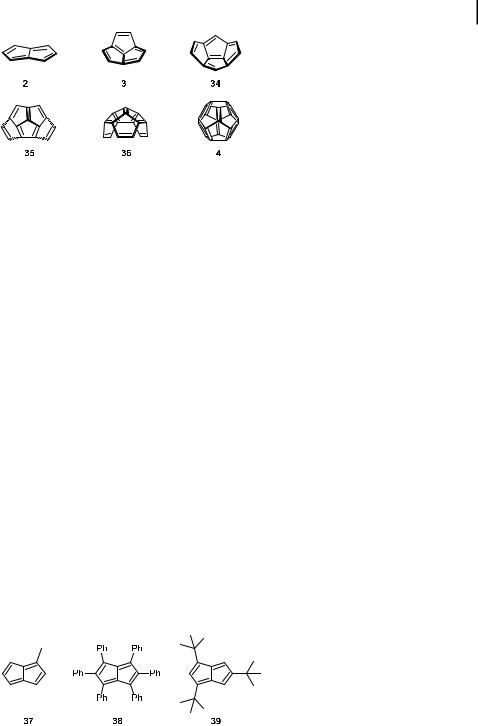
2.4 Polyunsaturated Di-, Tri-, and Oligoquinanes 39
quinanes 4, 34–36 have a considerable inherent curvature, which increases with an increasing number of annelated five-membered rings. This is confirmed by high level ab initio calculations (see below). In view of this, it can safely be assumed that 3 and higher vinylogues 4, 34–36, if accessible at all, would each have to be made from a precursor that bears close structural resemblance to the desired fully unsaturated oligoquinane.
2.4.1
Pentalene, Pentalenediide, and Pentalene Metal Complexes
Bicyclo[3.3.0]octa-1,3,5,7-tetraene (2), trivially called pentalene [26, 27], is the second member in the series of fully unsaturated oligoquinanes. Hu¨ckel MO theory predicts that this planar hydrocarbon with its 8p-electron system should be an antiaromatic species [25]. 2- Methylpentalene (37) has been generated by a retro-Diels–Alder reaction and deposited as a film at 196 C on a NaCl or quartz plate for its spectroscopic characterization. It rapidly dimerized upon warming the cold plates to temperatures above 140 C [26]. Only two stable derivatives of pentalene not complexed to a metal [29], the hexaphenyl- (38) [30] and 2,4,7-tri-tert-butylpentalene (39) [31], have hitherto been reported (Figure 4).
The question of aromaticity versus antiaromaticity and delocalized versus localized double bonds in pentalene (2) dates back to 1922, when Armit and Robinson compared it with naphthalene and postulated that the former might be similarly aromatic [32, 33]. While the first synthesis of a non-fused hexaphenylpentalene (38) [30] provided only some clues as to the non-aromatic reactivity of the pentalene skeleton, the tri-tert-butyl derivative 39, prepared and studied by Hafner et al. in great detail [31], gave a better insight. The ring-proton signals of this alkyl-substituted pentalene 39 are shifted upfield compared to those of fulvene (27) and other cyclic polyenes. This observation led to the conclusion that the pentalene derivative 39 should be an antiaromatic species. However, the results did not permit a distinction
Fig. 4. An unstable and two stable pentalene derivatives that have been observed experimentally.

402 Oligounsaturated Five-Membered Carbocycles -- Aromatic and Antiaromatic Compounds
to be made as to whether the double bonds in 39 are localized or whether they are subject to a rapid bond shift between the two Kekule´ structures. An indication that the parent system 2 has alternating bonds has been obtained by comparing the photoelectron (PE) and electronic absorption (UV/vis) data with calculated orbital energies [34]. More recent DFT calculations at the B3LYP/6-311þG*/B3LYP/6-31G* þ ZPVE (B3LYP/6-31G*) level of theory have revealed that pentalene (2) has a localized antiaromatic p-system with a singlet ground state lying 9.7 kcal mol 1 below the triplet state [35]. The calculated structural parameters of 2 and its dication 22þ (see Figure 5) appear to be quite reliable as the agreement between calculated and experimental bond lengths for pentalene dianion 22 and its dilithium derivative 22 2Liþ is excellent.
Brown et al. [36] attempted to approach pentalene (2) by a thermolytic ring-contraction– cyclization of 3-ethenylbenzyne 41, which, in turn, was generated by flash vacuum thermolysis of 3-ethenylphthalic anhydride 40. Indeed, the pentalene dimer 43 (@50%) was formed along with phenylacetylene 44 (@50%) in 80% overall yield (Scheme 6). The failure to detect monomeric pentalene (2) is in accord with the observation of de Mayo et al. that 1- methylpentalene (37) dimerizes above 140 C [29]. The formation of phenylacetylene 44 was unexpected, and it is as yet unclear as to whether it arises by migration of two hydrogens in the aryne 41 or the intermediate carbene 42, or whether it is a secondary product formed from pentalene (2).
Scheme 6.
Recently, the neutral pentalene (2) has been generated from its dimer 43 by photocleavage in an argon matrix [37]. In this structurally characterized cross-conjugated hydrocarbon 2, the distortive force of the p-electrons favors a C2h structure with localized single and double bonds, and this e ect predominates over that of the s-electrons, which would drive the molecule towards D2h symmetry (Figure 5).
Both the synthesis [38] and X-ray crystal structure [39] of dilithium pentalenediide 22 2Liþ have been reported (Scheme 7, Figure 5). Reaction of the dihydropentalene 46 with n-butyllithium yielded the crystalline dilithium pentalenediide 22 2Liþ (Scheme 7). The more recently reported flash vacuum thermolysis of 6-norbornenylfulvene (45), initially producing 6-ethenylfulvene, which immediately cyclizes to dihydropentalene (46), allows one to prepare this immediate precursor to 22 in gram quantities [40]. A similarly convenient

2.4 Polyunsaturated Di-, Tri-, and Oligoquinanes 41
Fig. 5. Calculated structural parameters of pentalene (2), pentalene dianion 22 (experimental values for dilithium pentalenediide 22 2Liþ in parentheses [39]), and pentalene dication 22þ.
Scheme 7.
access to the pentalene skeleton is by flash vacuum thermolysis of easily prepared ethenylbicyclopropylidene 47, which yields a 1:1.1 mixture of the tetrahydropentalenes 48 and 49 (90%) [41].
Structural analysis of the dilithium pentalenediide revealed a C2h-symmetric ion triplet with the two lithium cations located on opposite sides of the two fused rings. The structural parameters were extremely well reproduced by ab initio calculations [35] (see Figure 5). Both the experimental structural parameters and calculated magnetic susceptibility exaltation classify the 10p-electron species 22 as an aromatic compound. Apparently, the lithium counterions in 22 2Liþ do not exert any significant e ect on the bond lengths of the dianion 22 . On the other hand, the antiaromatic pentalene (2) and its aromatic dication 22þ show the characteristic bond length alternation (Figure 5) [35].
The first metal complex (50) of unsubstituted pentalene (2) was prepared in 1973 by reaction of the pentalene ½2þ2& dimer 43 with Fe2CO9 (Figure 6) [42]. Several other dinuclear complexes of 2 have been prepared from the dilithium pentalenediide 22 2Liþ [43]. In all
Fig. 6. Dinuclear pentalene complexes.

422 Oligounsaturated Five-Membered Carbocycles -- Aromatic and Antiaromatic Compounds
these complexes, the pentalene ligand is completely planar, and each five-membered ring is coordinated to a di erent metal atom either as in 50 or as in bis(pentamethylcyclopentadienylnickel)pentalene 51, which was obtained by reaction of 22 2Liþ with Cp*Ni(acac) [44].
More recently, Jonas et al. presented a new mode of complexation of the pentalene ligand, in which all eight carbon atoms are coordinated to only one metal atom (Scheme 8). Dilithium pentalenediide 22 2Liþ reacts with Cp2VCl to yield the vanadium pentalene-Cp complex 52 [45]. Reaction with Cp2ZrCl2 or Cp2HfCl2 gives the bis(pentalene) metal complexes 53 [45b]. In these complexes, all the carbon–metal bonds have similar lengths and the out-of-plane deformation angle of the pentalene skeleton is ca. 43 .
Scheme 8.
2.4.2
Acepentalene, Acepentalenediide, and Acepentalene Metal Complexes
In the series of fully unsaturated oligoquinanes, the tricyclic acepentalene (3) is the first member to exhibit a curved molecular surface, and – at least according to its number of carbon atoms – it resembles one hemisphere of the C20-fullerene (4). For almost five years this C10-bowl 3 remained the largest fully unsaturated oligoquinane ever observed [28]. According to Hu¨ckel MO theory, acepentalene (3) should have a triplet ground state [25], but more recently performed ab initio calculations have indicated the singlet state to be more stable by 3.9 kcal mol 1 [28]. The prohibitively high strain in the molecule, which by far exceeds that of pentalene (2), renders the isolation of 3 impossible at ambient temperature. As early as 1964, Woodward introduced the idea of using triquinacene 54 as a stable tricyclic precursor of acepentalene (3) [46]. However, it took another 30 years to generate acepentalene by the stepwise introduction of double bonds. The breakthrough came with the facile preparation of dipotassium acepentalenediide 32 2Kþ [47] and the purification trick of obtaining the pure dilithium derivative 32 2Liþ via the bis(trimethylstannyl)- dihydroacepentalene 56 (Scheme 9) [48]. The dilithium acepentalenediide 32 2Liþ permitted the first X-ray crystal structure determination of a bowl-shaped aromatic dianion; it was found to aggregate as a homodimer held together by two dimethoxyethane-ligated lithium counteranions in the solid state (Scheme 9). The immediate precursor to the dilithio derivative 32 2Liþ, the bis(stannyl)dihydroacepentalene derivative 56, is thermally stable and can be purified by sublimation [49]. However, the unsubstituted dihydroacepentalene 55 readily dimerizes in a ½4þ2& cycloaddition mode at ambient temperature, and it can only be observed as a monomer at temperatures below 80 C [49, 50].
The bis(stannane) 56 also proved to be a perfect precursor for the generation of the neutral acepentalene 3 [28]. By cleavage of the two labile tin–carbon bonds in 56 a diradical is