
- •Preface
- •Acknowledgments
- •Basic Physics
- •Transducers and Resolution
- •Doppler Physics
- •Artifacts
- •Summary
- •Introduction
- •Patient Preparation
- •Patient Positioning
- •Transducer Selection
- •Two-Dimensional Images
- •Two-Dimensional Imaging Controls
- •Introduction
- •M-Mode Echocardiography
- •Color-Flow Doppler
- •Spectral Doppler
- •Tissue Doppler Imaging
- •Measurement and Assessment of Two-Dimensional Images
- •Measurement and Assessment of M-Mode Images
- •Measurement and Assessment of Spectral Doppler Flow
- •Measurement and Assessment of Tissue Doppler Imaging
- •Evaluation of Color-Flow Doppler
- •Evaluation of Ventricular Function
- •Mitral Regurgitation
- •Aortic Regurgitation
- •Tricuspid Regurgitation
- •Pulmonary Regurgitation
- •Endocarditis
- •Pulmonary Hypertension
- •Systemic Hypertension
- •Hypertrophic Cardiomyopathy
- •Dynamic Right Ventricular Outflow Obstruction
- •Moderator Bands
- •Dilated Cardiomyopathy
- •Right Ventricular Cardiomyopathy
- •Restrictive Cardiomyopathy
- •Endocardial Fibroelastosis
- •Arrhythmogenic Right Ventricular Cardiomyopathy
- •Myocardial Infarction
- •Myocardial Contusions
- •Pericardial Effusion
- •Neoplasia as a Cause of Pericardial Effusion
- •Pericardial Disease
- •Abscesses
- •Pericardial Cysts
- •Thrombus
- •Ventricular Septal Defect
- •Patent Ductus Arteriosus
- •Aorticopulmonary Window
- •Right to Left Shunting PDA
- •Atrial Septal Defects
- •Endocardial Cushion Defects
- •Bubble Studies
- •Atrioventricular Valve Dysplasia
- •Outflow Obstructions
- •Inflow Obstructions
- •Tetralogy of Fallot
- •APPENDIX ONE Bovine
- •APPENDIX TWO Canine
- •APPENDIX THREE Equine
- •APPENDIX FOUR Feline
- •APPENDIX FIVE Miscellaneous Species
- •Index
has a velocity of 5 m/sec, the proximal chamber must have a pressure of at least 100 mm Hg (4 × 52) more than the right atrium. If right atrial pressure is 0 then the proximal chamber has a pressure of at least 100 mm Hg. Assuming normal right ventricular pressure in the distal chamber of about 20 mm Hg (barring any other congenital defect), the pressure gradient across the stenotic area can be estimated at around 80 mm Hg.
Cats with infundibular hypertrophy and obstruction almost all have evidence of small left ventricular chambers and left ventricular outflow obstruction with left ventricular infundibular hypertrophy and evidence of systolic anterior mitral valve motion in some. The cause of the LVOT obstruction is not clear. It is hypothesized that it may be hypertrophic cardiomyopathy or volume contraction (84). Cats with severe infundibular obstruction to outflow had right ventricular outflow tract diameter to pulmonary valve diameter ratios of <.25.
This defect is easily missed or can be confused with a ventricular septal defect. The turbulent flow associated with a midventricular obstruction on transverse right parasternal imaging planes is close to the same location that shunted blood from a ventricular septal defect enters the right ventricular chamber. It can also be misdiagnosed as valvular pulmonic stenosis if a CW Doppler cursor is partially aligned with the midventricular turbulent jet. Use structural changes within the right side of the heart to help identify the presence of a possible midventricular or infundibular obstruction. An abrupt decrease in hypertrophy within the right ventricular chamber or outflow tract should be a clue that a midventricular or infundibular obstruction exists and use color-flow Doppler to help identify the origination of turbulence (91,93).
Inflow Obstructions
Mitral Stenosis
Mitral stenosis is a rare congenital abnormality in dogs and cats. It has a high incidence in the Bull terrier and Newfoundland (94–98). It may occur as a single entity or in combination with other defects. In man, it is often found in conjunction with subaortic stenosis, patent ductus arteriosus, ventricular septal defect, endocardial fibroelastosis, and coarctation of the aorta. Concomitant defects in dogs and cats include subaortic stenosis, patent ductus arteriosus, pulmonic stenosis, and mitral valve dysplasia (95,96,98,99). Mitral stenosis in man is usually acquired secondary to endocarditis, rheumatic fever, or a variety of other causes, but its development as an acquired lesion in animals cannot be confirmed (96).
Mitral stenosis has varying morphologies, which may include thickened leaflets with hypertrophied papillary muscles, a supravalvular ring, billowing leaflets with abnormal chordae all attached to one papillary muscle creating a tunnel, and hypoplastic valves (95,96,99). The diagnosis of mitral stenosis was the first clinical application of ultrasound in man, and the disease has some very characteristic echocardiographic findings.
M-mode echocardiography shows thick mitral leaflets, parallel motion of the anterior and posterior mitral valve leaflets (concordant motion), prolonged mitral valve EF slope, and a dilated left atrium (Figure 10.41). Two-dimensional findings include a dilated left atrium, doming of the mitral valve leaflets, reduced excursion of either or both mitral leaflets, thick mitral valve leaflets, and possibly a supravalvular ring (Figures 10.42, 10.43, 10.44). A pressure gradient, increased pressure half-time, and possibly mitral insufficiency are present on Doppler examination of mitral stenosis (94–97,99).

Features:
Mitral Stenosis
Concordant MV motion
Doming of MV leaflets
Reduced EF slope
Prolonged PHT
High PG
Dilated LA
Figure 10.41 M-mode echocardiography shows thick mitral leaflets, concordant motion of the anterior and posterior mitral valve leaflets (arrow), and prolonged mitral valve EF slope in hearts with valvular mitral stenosis. RV = right ventricle, LV = left ventricle, MV = mitral valve.
Figure 10.42 When the tips of the mitral valves point toward each other (arrows) as opposed to pointing toward the left ventricular chamber during maximal mitral valve excursion the leaflets are said to dome, a sign of mitral stenosis. Plane = left parasternal apical five-chamber view, LV = left ventricle, LA = left atrium, AO = aorta, MV = mitral valve.

Figure 10.43 Doming of the mitral valves may be seen on parasternal long-axis views as well. The leaflet tips point toward each other at maximal excursion implying fusion of the valve leaflets. Both leaflets dome (arrows). The left atrium is dilated secondary to back pressure and possibly insufficiency. Plane = right parasternal long-axis four-chamber view, LV = left ventricle, LA = left atrium.
Figure 10.44 Mitral stenosis may manifest as a supravalvular ring (large arrow). The mitral valves are normal (small arrow). Plane = right parasternal long-axis four-chamber view, LV = left ventricle, LA = left atrium.

M-Mode Evaluation
Concordant mitral valve motion means the leaflets move in parallel; the posterior and anterior leaflets do not move away from each other as they normally do. The posterior leaflet will move upward toward the septum at the same time the anterior leaflets does. This is seen when there is commissural fusion of the leaflets and is easy to document on M-mode images (Figure 10.41).
Two-Dimensional Evaluation
Two-dimensional images show doming of the mitral valve leaflets when there is fusion of the cusps (Figures 10.42, 10.43). The tips of the leaflets will point toward each other at maximal opening. The body of the anterior leaflet will bow or dome up toward the outflow tact, and sometimes the posterior leaflet domes toward the free wall of the left ventricle.
Fusion of the mitral leaflets also reduces the anterior and posterior excursion of leaflets during diastole. In order to document the reduced excursion during diastole, look at the tips of the leaflets and not along the body of the leaflets where the septal leaflet will billow up toward the outflow tract and the parietal leaflet billows down to the free wall. To document this on M-mode images, the M- mode cursor must be positioned at the tips of the leaflets. The mitral valve EF slope will be reduced.
Although the finding of concordant motion is diagnostic of mitral stenosis, the latter two findings— reduced EF slope and reduced excursion—are not specific for the disease. Reduced excursion may be seen in the presence of aortic insufficiency, a common finding in dogs with subaortic stenosis. Almost one-half of dogs with mitral stenosis in one report also had subaortic stenosis (96). Poor flow into the left ventricle for any reason will also reduce mitral valve motion and excursion. Doming of the body of the leaflets will differentiate reduced motion secondary to aortic insufficiency or reduced flow from poor excursion secondary to mitral stenosis. Reduced ventricular compliance may be a cause of delayed early diastolic mitral valve closure. This is often seen with heart disease resulting in left ventricular hypertrophy. There are also reports of mitral stenosis in man in which concordant mitral valve motion is not present or may not be evident (95,99,100).
A thick mitral valve is usually present with mitral stenosis but may also be seen with dysplastic valves, subaortic stenosis, and acquired degenerative or infective lesions. The diagnosis of mitral stenosis as opposed to these other differentials can be made when the other features of stenosis are present including doming, reduced EF slope, concordant diastolic motion, and increased pressure half-

time (95).
Restriction to left ventricular filling causes left atrial pressure to increase and the left atrium to dilate (Figure 10.43). Elevation in pressure secondary to increased output due to excitement or exercise may exacerbate the clinical signs associated with mitral stenosis. The development of atrial arrhythmias especially atrial fibrillation will decrease flow into the ventricle and elevate atrial pressure. The degree of left atrial dilation is affected not only by the obstruction to left ventricular inflow but also by the degree of mitral insufficiency if present (94,96).
Chronic elevation in left atrial and pulmonary venous pressure will lead to chronic interstitial changes within the pulmonary arterioles and secondary pulmonary hypertension. Pulmonary hypertension affects the right side of the heart, and echocardiographic evidence of pulmonary hypertension includes right ventricular and pulmonary artery dilation, right ventricular hypertrophy, tricuspid insufficiency, and changes in the pulmonary flow profile. The echocardiographic findings in pulmonary hypertension are discussed in Chapter 6. When hypertension becomes significant, decreases in left ventricular preload may alleviate some of the symptoms of mitral stenosis (101).
Mitral valve area in man is measured directly from transverse images of the mitral valve. Normal mitral valve area in the dog is 3.69 ± 1.42 cm2 (Figure 10.45) (102). An area greater than 2.5 cm2 (normal 4–6) in man typically creates only mild symptoms. A valve area of less than 1 cm2/m2 of body surface area represents severe mitral stenosis in man. This has not been validated in animals. Problems with direct measurement include poor resolution, improper selection of the frame for measurement, and gain settings. When the transverse image is not perpendicular to the mitral valve and an oblique view is used, the size of the mitral orifice may be incorrectly assessed. The tips of the mitral valve leaflets must be seen in cross section otherwise the orifice size will be overestimated secondary to billowing of the body of the leaflets.
Mitral Valve Area
Canine—normal
3.69 ± 1.42 cm2
Figure 10.45 Mitral valve area is measured directly from transverse images of the mitral valve. Normal mitral valve area in the dog is 3.69 ± 1.42 cm2. Plane = right parasternal transverse mitral valve, RV = right ventricle, LV = left ventricle, VS = ventricular septum, MVA = mitral valve area.

Color-Flow Doppler Evaluation
The narrow mitral orifice secondary to commissural fusion of the mitral valve creates an aliased color jet flowing into the left ventricle during diastole representative of increased velocity and turbulence (Figure 10.46). When aortic valve or outflow tract pathology is also present, be sure to differentiate this mitral jet from diastolic aortic insufficiency within the outflow tract. Systolic aliased signals may also be seen since many of these patients also have mitral insufficiency (95,96).
Figure 10.46 Color-flow Doppler shows a narrow aliased signal during diastole when mitral stenosis is present (arrow). There is flow acceleration on the left atrial side of the stenosis. Plane = left parasternal apical five-chamber view, LV = left ventricle, LA = left atrium, MV = mitral valve, RV = right ventricle, AO = aorta.
Spectral Doppler Evaluation
Spectral Doppler of mitral inflow shows high velocity flow profiles with reduced early diastolic slopes or lack of closure during early diastole. The inflow profile is recorded from apical fouror five-

chamber views of the heart. The reduced slope reflects delayed normal early diastolic closure because of a persistent pressure differential between the left atrium and left ventricle (Figure 10.47). Measurement of the slope provides a calculation of pressure half-time. Pressure half-time is the time required for pressure to decrease to one-half its peak value. Normal pressure half-times in the dog are 29 ± 8 msec (<50 msec) and are reported to be <30 msec in the cat (95,96,99,103). The longer the pressure half-time, the more severe the stenosis. Normally during early ventricular filling, the left ventricular pressure rises and left atrial pressure decreases resulting in a rapid deceleration of the E peak on transmitral flow profiles when the pressure gradient between the two chambers rapidly equalizes. With mitral stenosis, the more severe the restriction to left ventricular inflow, the longer the pressure gradient is maintained between the two chambers and the slower the decline of the MV E peak pressure during early diastolic filling. Pressure half-times in mitral stenosis usually exceed 90 msec in man and may be much higher. Reported pressure half-times exceed 80 msec in cats and ran to 330 msec in dogs with mitral stenosis (95,96,99).
PHT and MS
Increased PHT
= persistent PG between LV and LA
Normal PHT—canine
29 ± 8 msec (<50 msec)
Normal PHT—feline
<30 msec
Figure 10.47 Spectral Doppler shows a reduced rate of mitral valve closure resulting in a long pressure half-time when mitral stenosis is present. The pressure half-time is used to calculate valve area. The mitral valve half-time (MV PHT) here is 190 msec and the valve area is 1.2 cm2.
The severity of stenosis correlates directly with pressure half-time (PHT). The longer the PHT, the more severe the stenosis is. Pressure half-time is affected by a variety of other factors including the presence of aortic insufficiency and irregular heart rhythms (95,104). The velocity across the stenosis is directly dependent upon left ventricular pressures. In the presence of aortic insufficiency, left ventricular diastolic pressure increases more rapidly secondary to this second source of blood volume,

especially if the insufficiency is acute, and pressure half-time will decrease correspondingly despite the presence of possibly severe mitral stenosis. When measuring pressure half-time, follow the actual outline of E to F slope. If the slope has a curve, follow the curve while tracing the slope. The A wave should also be ignored if present, a sweep speed of 100 mm/sec should be used for greater accuracy, and average at least 3 beats. If the animal is in atrial fibrillation or has another irregular rhythm, average the half-times of 5 to 10 beats (105).
Mitral valve area can be calculated using pressure half-time (PHT) (Figure 10.47). This information is used to determine the timing of surgical intervention and is of lesser value at this time in animals. Apply Equation 10.5 to calculate an orifice area:
Equation 10.5
where PHT is measured in msec, and the area is reported as cm2 (94,106,107).
Mitral stenosis creates an obstruction to inflow that results in elevated left atrial pressures. The Bernoulli equation can be applied to peak or mean mitral inflow velocities to determine the pressure gradient. The more restrictive the stenosis, the higher the gradient. Mean pressure gradients are considered more accurate in the assessment of severity of mitral stenosis. The flow profile is traced, and mean gradients are calculated by the equipment (Figure 10.48). Some studies have even shown that Doppler-derived gradients for mitral stenosis are more accurate than invasive catheterization methods. Normal dogs should have a peak mitral valve E velocity of 1.1 and pressure gradients less than 5 mm Hg between the left atrium and left ventricle during diastole (56,96). A peak gradient greater than this suggests the presence of mitral stenosis (Figure 10.49).
Mitral Stenosis
Mean PG more accurate than peak PG
Figure 10.48 A pressure gradient is calculated from the mitral inflow profile. A mean gradient is more accurate than peak gradient. A gradient greater than 5 mm Hg suggests obstruction. The mean gradient (meanPG) here is 6.56 mm Hg and the peak gradient (maxPG) is 14.2 mm Hg. MV = mitral valve, Vmax = maximal velocity, Vmean = mean velocity, HR = heart rate.
Figure 10.49 Doming of thick abnormal tricuspid valves (arrow) with commissural fusion is seen in

this dog with tricuspid stenosis. This dog also had an atrial septal defect, not seen in this image. Plane = right parasternal long-axis four-chamber view, RV = right ventricle, RA = right atrium, LV = left ventricle, LA = left atrium.
Pressure gradients for any given orifice area however are influenced by the amount of volume flowing through it. For any given mitral valve area, larger volumes will increase the pressure gradient. Therefore, animals with mitral stenosis and significant mitral insufficiency will have higher pressure gradients than animals with the same valvular area and little or no mitral insufficiency. Similarly, low flow states will underestimate the pressure gradient. Elevations in left ventricular diastolic pressure secondary to aortic insufficiency or poor left ventricular compliance will also reduce the derived gradient for any given stenotic area.
Radial left ventricular wall motion has been assessed with color tissue Doppler imaging in a dog with mitral stenosis (94). No abnormalities from normal were found in this Bull terrier suggesting that systolic and diastolic function were normal. There was also no discordant motion between the endocardium and epicardium.
It is obvious that any one given feature of mitral stenosis cannot be used to make the diagnosis. A combination of features plus characteristic real-time images consistent with reduced mitral valvular motion, doming, thickened leaflets, abnormal chordae, supravalvular rings, etc., will provide accuracy in diagnosing the presence of mitral stenosis correctly even if the assessment of severity is sometimes not possible.
Tricuspid Stenosis
Isolated congenital tricuspid stenosis is uncommon, and there are rare reports of it in a Labrador retriever and a Chesapeake Bay retriever in veterinary medicine (108–110). Tricuspid stenosis in conjunction with tricuspid dysplasia is seen more often. Tricuspid stenosis with patent ductus arteriosus and pulmonic stenosis is described in an Arabian foal (111).
Two-Dimensional and M-mode Evaluation
The features of tricuspid stenosis are similar to those seen with mitral stenosis. There is doming of the

leaflets, reduced excursion of the valve tips, concordant motion of the anterior and septal leaflets, a dilated right atrium, and often, tricuspid insufficiency. The free edges of the valve are apically displaced, but the annulus is not displaced (Figure 10.49) (108,109). The right ventricle will be dilated if there is tricuspid insufficiency but will be normal or small if there is no valvular regurgitation. The tricuspid valve may be nodular, thick, and can be dysplastic with short chordae tendinae and abnormal papillary muscles (Figure 10.50). Right to left shunting through a patent foramen ovale may exist when right atrial pressure is high enough.
Features:
Tricuspid Stenosis
Concordant TV motion
Doming of TV leaflets
Reduced EF slope
Prolonged PHT
High PG
Dilated RA
Possible patent foramen
Figure 10.50 A stenotic tricuspid valve may be nodular, thick, and can be dysplastic with short chordae tendinae and abnormal papillary muscles (arrows). Plane = foreshortened left apical fourchamber view, RV = right ventricle, LV = left ventricle, TV = tricuspid valve, RA = right atrium.
M-mode images show paradoxical septal motion where the septum move down toward the left ventricular chamber during diastole secondary to elevated diastolic pressure in the right side of the heart relative to the left. The parietal leaflet of the tricuspid valve will show slow deceleration time because of delayed equilibration of pressure between the right atrium and ventricle. Normally right ventricular pressure increases rapidly and right atrial pressure decreases rapidly during early diastole giving the E peak of tricuspid valve motion a rapid deceleration time (110). The left ventricular chamber may be smaller than normal because of poor right ventricular forward stroke volume if the animal is in right-sided congestive heart failure and because of the paradoxical septal motion (110).

Color-Flow and Spectral Doppler Evaluation
Color-flow Doppler shows turbulent flow passing through a visibly small orifice. The turbulent signal starts at the tips of the tricuspid valve leaflets during diastole (Figure 10.51). There is no turbulence during systole unless the valve is also insufficient. Spectral Doppler shows high velocity flow greater than 1.0 m/sec and slow deceleration during early diastole resulting in a long pressure half-time (Figure 10.52). Use color-flow Doppler to define the imaging plane that aligns the Doppler cursor along the right ventricular inflow pathway. This imaging plane may be the left apical fouror fivechamber view, a modification of the apical view, or a right parasternal transverse plane (108–110).
Figure 10.51 (A) Color-flow Doppler shows turbulent flow passing through a small tricuspid orifice. The turbulent signal and flow acceleration starts at the tips of the tricuspid valve leaflets during diastole. (B) There is no turbulence during systole unless the valve is also insufficient as it is here. Plane = foreshortened left apical four-chamber view, RV = right ventricle, LV = left ventricle, LA = left atrium, TV = tricuspid valve, RA = right atrium.
Figure 10.52 (A) Spectral Doppler shows high velocity flow of 3.1 m/sec and a mean pressure gradient of around 13 mm Hg and (B) slow deceleration during early diastole resulting in a long

pressure half-time (238 msec) in this heart with tricuspid stenosis. TV = tricuspid valve, Vmax = maximum velocity, Vmean = mean velocity, max PG = maximum pressure gradient, mean PG = mean pressure gradient, VTI = velocity time integral, HR = heart rat.
Peak flow velocity is increased in order to maintain normal flow volume through the valve (based upon the continuity equation) and is proportionate to the degree of stenosis. Deceleration of flow during early diastole will be slow because of the increased time for right ventricular and right atrial pressure to equalize (108–110,112–114). If right atrial pressure is not very elevated for instance then it would not take long for the pressure gradient between the atrium and ventricle to diminish as in normal transvalvular flow where we see a rapid deceleration rate of flow during early (E wave) diastole. The more stenotic and restricted flow is through the valve, the higher the right atrial pressure and the longer it will take a pressure gradient to diminish.
Peak trans tricuspid flow velocity increases in response to the high right atrial pressure and the effort to maintain normal forward flow. The increased flow velocity is proportionate to the severity o f the stenosis. Pressure in the right side of the heart is low, and even a mean pressure gradient of 5 mm Hg in humans suggests the presence of hemodynamically significant tricuspid stenosis (108). Mean pressure gradient is calculated by tracing the flow velocity integral (Figure 10.52).

Tricuspid Stenosis
Mean PG in man >5 mm Hg = significant TS
Cor Triatriatum
Cor triatriatum refers to the division of the right or left atrium into cranial and caudal cambers. The membrane on the right side is a remnant of the embryonic right sinus venosus valve. When the membrane is present within the right atrium, it is referred to as cor triatriatum dexter (115–117). Its presence within the left atrium is referred to as cor triatriatum sinister, and it is the result of improper resorption of the common pulmonary veins during embryonic development (118). Cor triatriatum dexter is typically found in the dog, while cor triatriatum sinister is primarily found in cats (115–117). Although cor triatriatum may be the only defect present, associated problems include tricuspid valve anomalies, pulmonic stenosis, patent ductus arteriosus, patent foramen ovale, mitral regurgitation, and persistent left cranial vena cava in man and in dogs (117–123). The patent foramen may shunt right to left if it is located within the high pressure chamber of cor triatriatum dexter.
Cor Triatriatum Dexter
Canine
Cor Triatriatum Sinister
Feline
Cor Triatriatum Dexter
Echocardiographic images of the right atrium show a membrane dividing the chamber into two (Figure 10.53). This membrane restricts venous return into the right side of the heart by obstructing caudal vena cava flow and possibly coronary sinus flow. The membrane varies in its location within the right atrium in man. The cranial chamber may receive either the caudal and cranial vena cava or only one of the vena cava. The tricuspid valve may be within either chamber depending upon the site of the membrane and its angle through the atrium. The majority of dogs have a low pressure distal chamber that receives the cranial vena cava and contains the tricuspid valve, and a proximal high pressure chamber that receives the caudal vena cava and the coronary sinus (123). The tricuspid valve is located within the distal chamber and may or may not be normal (116, 117,124–126). Atypical angles and planes through the right atrium are sometimes necessary in order to visualize the membrane. The membrane may also form a tunnel type of connection between the cranial and caudal chambers as opposed to forming a distinct division into two chambers.
Figure 10.53 (A) A membrane divides the right atrium into a cranial (proximal) high pressure chamber and caudal (distal) low pressure chambers when cor triatriatum dexter exists. (B) The connection (arrow) between the two parts of the right atrium may not be obvious without color-flow Doppler. Plane = right parasternal transverse heart base, RA = right atrium, TV = tricuspid valve, RV = right ventricle, LA = left atrium, IAS = interatrial septum, AO = aorta, M = membrane.
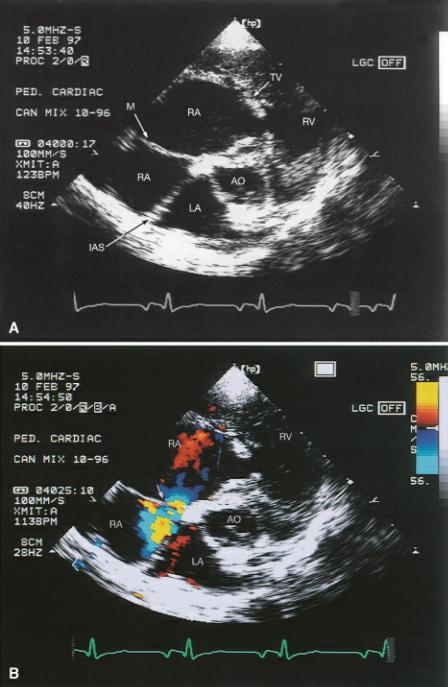
The perforation, which allows blood to flow from the cranial to the caudal chamber, varies in size and the degree of obstruction to flow. It is often not apparent without color Doppler echocardiography. The membrane may be seen on right parasternal transverse or four-chamber views that show the vena cava as well as on apical fourand five-chamber views. Rotate and fan the transducer in multiple directions in order for the sound beam to interrogate all aspects of the right atrium (116, 117). Spectral or color-flow Doppler can confirm the presence of obstruction. A pressure gradient between the two chambers will exist, and turbulent flow can be documented. A diffuse membrane with many fenestrations, the Chiari network, is a variant of this defect, and in most cases this is not hemodynamically significant (124). The cranial chamber and vena cava will dilate if a significant obstruction to flow into the right side of the heart exists. The rest of the heart may appear perfectly normal. Any young animal with signs of right-sided heart failure should be examined thoroughly for cor triatriatum dexter. As an isolated defect, no murmur is typically heard.
Cor Triatriatum Sinister

In cor triatriatum sinister, the membrane divides the left atrium into cranial and caudal chambers (Figure 10.54). This membrane should be differentiated from supravalvular mitral stenosis. The fibrous ring associated with mitral stenosis is always distal to the fossa ovalis, closer to the mitral valve and inferior to the left auricular appendage. The membrane of cor triatriatum sinister is located proximal to the fossa ovalis near the base of the atrium. The left auricular appendage is in the low pressure compartment of cor triatriatum sinister (127). The pulmonary veins typically empty into the proximal chamber (high pressure chamber), and the distal chamber (low pressure chamber) contains the mitral valve and left auricular appendage (128).
Cor Triatriatum Sinister
Membrane
Proximal to fossa ovalis
Left auricular appendage
In low pressure chamber
Distal to membrane
Supravalvular Mitral Stenosis
Membrane
Distal to fossa ovalis
Left auricular appendage
In high pressure chamber
Proximal to membrane
Figure 10.54 (A) A membrane separates the left atrial chamber in cranial and caudal sections with cor triatriatum sinister. (B) Spectral Doppler reveals a pressure gradient of 35 mm Hg between the cranial and caudal chambers of the left atrium. Plane = right parasternal four-chamber view, LV = left ventricle, LA = left atrium, IAS = interatrial septum, M = membrane, MV = mitral valve.

Cor triatriatum sinister can be subcategorized into three types depending upon the shape and location of the membrane and its relationship with the pulmonary veins and interatrial septum. Type A cor triatriatum has a funnel-like membrane that connects the proximal and distal chambers. The pulmonary veins in this type of membrane may all enter the proximal chamber (high pressure), be divided between the two chambers, or there may be anomalous drainage into the right atrium. Type B cor triatriatum has a wedge-shaped membrane with the fossa ovalis located within the proximal chamber. Pulmonary veins may all enter the proximal chamber and blood shunts to the right atrium, or they may be divided between both portions of the atrium. Type C cor triatriatum sinister involves a midline membrane where the foramen ovale is located in the proximal chamber with all pulmonary veins entering the distal low pressure chamber. The type and location of the membrane plays a large role in resulting clinical signs and development of congestive heart failure (129). Doppler can confirm the presence of a pressure gradient. Significant gradients may lead to left heart failure and pulmonary hypertension.